When Earth is seen from the depths of space it appears as a blue dot. This is because just over 70 per cent of its surface is covered with water. Water is present on many of the planets too, and several moons of Jupiter and Saturn are thought to have significant water resources. But Earth is, without doubt, the one body in our Solar System where water has a defining presence. It’s thanks to water and its physical peculiarities that life has flourished in the first place. Water is so familiar to us that we often forget just what a remarkable substance it is.
What exactly is water?
Water is H2O, a simple molecule consisting of two atoms of hydrogen and one of oxygen. It is the only substance that exists as solid, liquid and gas in the temperature ranges found naturally on Earth. Water is transparent, but isn’t entirely colourless. Just as the sky is blue because molecules in the atmosphere scatter blue light more than other colours, so large quantities of water have a similar blue tinge, whether it’s the ocean or the dramatic blues of glacier ice (oceans and lakes also reflect a blue sky, making them appear even bluer). We are extremely lucky to have so much water on Earth because it has remarkable properties.

What’s so special about it?
Water is an impressive solvent, which means it is extremely good at dissolving things. This is partly why it’s so valuable for living organisms, acting as a transport fluid for a whole host of chemicals in living cells. What makes water such a good solvent is its ability to stick onto and separate the atoms of a substance, which is thanks to unusually strong hydrogen bonding. This is the effect that makes water so special: an electrical attraction between hydrogen atoms and other atoms such as nitrogen, oxygen, and fluorine. Hydrogen bonding between water molecules also makes them hard to separate, pushing up the boiling point. Without this effect, water would boil at around -70°C. That would mean no liquid water on Earth – and no life.
Another essential side effect of hydrogen bonding is that when water freezes, the hydrogen bonds between the molecules pull the crystals into a particular shape. This is why snowflakes form with six points, and it means that water crystals have more space in them than they otherwise would. They form tetrahedrons – shapes with four triangular sides. As a result, solid water, or ice, is less dense than the liquid form, which is why it’s not recommended to put a glass bottle of water in the freezer (the water will expand and can shatter the bottle), and why ice floats on a pond.
It’s often said that this property of water is unique. This is not quite true, as acetic acid and silicon, for example, are both less dense as a solid than as a liquid. But it is unusual, and it’s important. If ice were denser than water, lakes would freeze from the bottom, not the top, making it far less likely that aquatic life could survive cold winters.

Where in the Universe have we found water?
The chemical elements making up water (hydrogen and oxygen) are plentiful in the Universe. In fact, they’re the first and third most common by mass. Therefore, it’s not surprising that water shows up in many places. Every planet in the Solar System has at least some water, though the furnace-like Venus only has tiny amounts of vapour in its atmosphere.
Similarly, some moons are well-provided. Our own Moon appears to have ice deposits, while a number of the moons of Jupiter and Saturn, such as Europa, Ganymede, Callisto and Enceladus, are thought to have salty liquid water under surface ice. Comets, which plunge towards the Sun from the outer Solar System, usually contain large amounts of water ice. Further out, we find water in vast clouds of material between the stars, in the atmosphere of planets in distant solar systems and in the rotating discs of matter where new stars are forming. Water is indeed common, though rarely as dominant as it is on Earth.
How do we know there’s water out there?
We can hardly go out to distant star systems and check for water, but astronomers have tried and tested methods to detect molecules in space. These rely on spectroscopy, or the study of the spectrum of light. When light passes through a material, some of the wavelengths of light get absorbed, leaving dark lines on the spectrum. Spectroscopy was first used in astronomy to detect elements in stars, but it is now widely used when light passes through, say, a cloud of matter in deep space.
Different compounds have their own distinct ‘absorption spectra’, like a fingerprint for a specific molecule. There are even distinctions, for example, between the spectra of liquid water and water vapour (though as yet we can’t detect liquid water on a planet unless we can observe it directly).
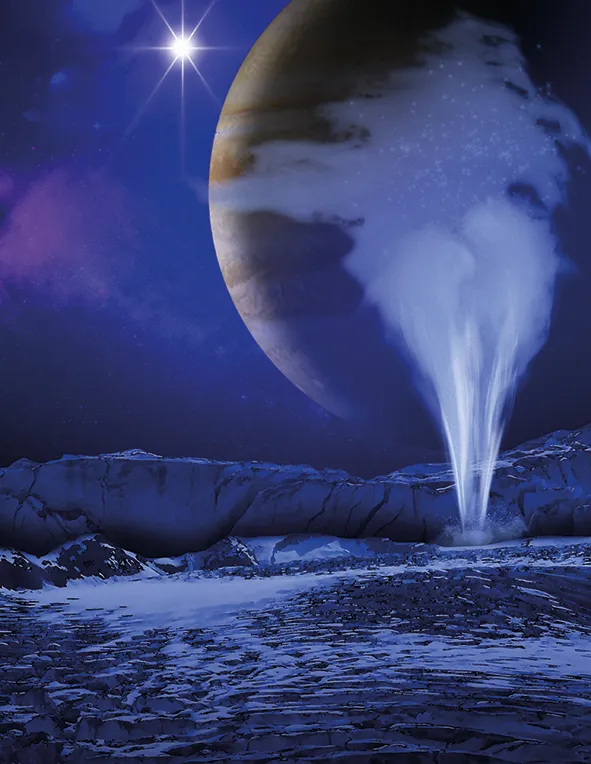
Detecting water vapour in the atmosphere of a planet orbiting a distant star is more difficult than detecting standalone water molecules in space, because the signal is harder to distinguish from the star’s own spectrum. However, a new technique being trialled by the European HotMol project combines spectroscopy with information about the light’s polarisation, which may give an indication of the spectrum’s source. Technology is increasingly making it possible to discover just how widespread water is in the Universe.
Is water essential for life?
Water is certainly essential to the forms of life we have on Earth, which all have a common ancestry and require water to function. Life has been found in all kinds of extreme environments, operating at the limits of heat and cold and even without air. But every type of life we have discovered contains biological cells that require water to provide their operating environment. Cells simply could not function without it. It’s not just a matter of keeping them inflated and moving chemicals around – living cells are full of tiny complex mechanisms. Many of these mechanisms rely on different sections of molecules either working well with water, or not mixing with it. Proteins, for example, are the key worker molecules in living organisms. Proteins have to fold into particular shapes to carry out their roles, and it is the interaction with water by various parts of a protein molecule that tell it how to fold. Water isn’t just a solvent: it is intimately involved in the functioning of our magnificent cellular machinery.
How much water do we have in our bodies?
We humans contain a large amount of water, typically between 50 and 70 per cent by weight. The majority of this is in the approximately 30 trillion cells that make up the body, while the rest is in fluids such as blood.

In our cells, water prevents the cell from collapsing and also acts as a medium for various molecules to get from place to place. This transport role is more obvious where the water is moving, such as carrying material around the bloodstream. It also lubricates, dissolves valuable chemicals, and acts as a shock absorber for organs. It’s hard to find a part of the body where water doesn’t play a role – even bones are around one-third water. Incidentally, although we do need about two litres (eight glasses) of water a day, we don’t need to drink it in its pure form, as other drinks can hydrate us too. Plus, we typically get around half our liquid requirements from water in food.
How did the Earth’s water get there?
Although we’re not 100 per cent certain of the origin of the Earth’s water, it’s generally thought to be a combination of water from the cloud of matter that initially formed the Earth, along with additional water from bodies that collided with the planet later. Being relatively close to the Sun, our planet would have lost some of its initial water during its formation, but bodies that are further out, such as asteroids and comets, were more likely to hold onto their water and could have added to the Earth’s supplies when the Sun’s gravitational pull brought them towards us.
For a long time, it was thought that a high percentage of the Earth’s water came from these later additions, but recent research shows that water on most comets and asteroids tends contain more deuterium – a hydrogen isotope – than water on Earth does. This suggests that a lot of the water on our planet dates back to the origin of the Solar System and that much of it might have been protected in the Earth’s early days by remaining well below the surface. In 2016 it was discovered that rocks at depths of up to 1,000 kilometres could store water.
How does the water cycle work?

Thanks to the Sun’s constant stream of energy, water molecules are continually evaporating as water vapour from the surfaces of oceans and lakes (as well as plants and soil), adding to the water vapour in the air. This is carried by winds around the planet. Where the vapour reaches particularly cold air, and has particles to condense around, it forms the tiny water droplets that make up clouds, which combine to form larger drops and eventually fall as rain. When rain falls on high ground, it runs down as streams and rivers, eventually feeding back into the oceans. This cycle is essential for the many living things that otherwise live on dry land.
Why do we have to save water when there’s so much of it?
One thing is definite. As a planet, we aren’t short of water. Over 70 per cent of the Earth’s surface is water – 1.4 billion cubic kilometres of the stuff. This is such a huge amount, it’s difficult to visualise. A cubic kilometre is a trillion litres of water. Divide the amount of water in the world by the number of people and we end up with 0.2 cubic kilometres of water each. With a reasonable consumption of five litres per person per day, the water in the world would last for 116,219,178 years. And that assumes that we use up the water. In practice, the water we ‘consume’ soon becomes available again for future use. Water shortages are really energy shortages – it’s the cost of energy that makes it difficult to provide usable water. The problem is that water is either in the wrong place, so needs moving, or needs something removing to make it drinkable – and nowhere is this more obvious than with seawater.

Why is it so difficult to convert seawater into drinking water?
Living in the UK, surrounded by ocean, it can seem absurd that we ever experience water shortages. The same applies to many countries with coastlines. And it is perfectly possible to convert seawater to drinking water. It’s simply an expensive and energy-intensive process.
Seawater typically contains around 3.5 per cent minerals by weight, mostly the sodium and chloride ions that form salt when the water is evaporated. The easiest way to make this drinkable is simply to boil the water and collect the pure vapour. Alternatively, hydrogen and oxygen can be separated from the seawater by electrolysis and recombined to make water, or the minerals can be removed by special membranes which only allow some molecules through. Practical desalination plants tend to use variants on the evaporation technique: the process isn’t difficult, it’s just that it takes a great deal of energy to remove the impurities, usually significantly less energy than is required to get water from other sources such as the ground or recycling.
This article first appeared in issue 305 of BBC Focus magazine - subscribe here.