It’s impossible to miss the hype around CRISPR – a new form of genetic modification that promises to revolutionise the field of gene therapy. Unlike older forms of genetic manipulation which are based on adding or swapping whole sections of DNA, CRISPR is a technique known as gene editing, allowing scientists to make precise changes in the genome down to the level of a single DNA ‘letter’.
In recent years we’ve seen academic and commercial researchers racing to get gene editing working in everything from tiny nematode worms and lab-grown cells to plants, animals and even humans. So how can we expect to see this powerful technology being used in the not-too-distant future?
Most of the excitement around CRISPR centres on its potential for treating human diseases, especially life-threating disorders caused by a fault in a single gene. In 2016, researchers at the Salk Institute in California used CRISPR to modify cells in the eyes of rats with a genetic form of blindness, partially restoring their sight – the first time the technique had been shown to work in adult mammals. Since then there has been promising progress towards gene editing therapies for a huge range of illnesses, from inherited metabolic problems and hereditary heart failure to cystic fibrosis and conditions affecting the brain, such as Huntington’s disease or even (more controversially) autism.
Because it’s tricky to deliver CRISPR into cells throughout the body, researchers have their sights set on relatively easy-to-access tissues such as the blood, skin, muscle and liver – for example, using CRISPR to convert liver cells into miniature ‘protein factories’, pumping missing molecules back out into the body. Another intriguing idea on the horizon involves using CRISPR to edit a sample of skin cells in the lab, growing them into a personalised skin patch for delivering hormones or other biological molecules.
Read more 2019 science breakthroughs:
Muscle-wasting disorders are also a target. Although the faulty gene responsible for Duchenne muscular dystrophy is very large, making it tricky to repair with CRISPR, scientists in Texas announced in August that they had managed to edit a ‘hotspot’ within the gene in beagle puppies with the disease – a fix that could work for up to 15 per cent of patients if the results translate into human trials.
Infectious diseases are another development on the horizon. In May, Japanese researchers used CRISPR to cut hidden HIV viruses out of the genomes of infected human immune cells growing in the lab. Unlike conventional anti-viral drugs, which control the virus but don’t get rid of it entirely, the development of a CRISPR-based therapy paves the way for the complete eradication of the disease from infected carriers.
Gene editing on trial
Despite these exciting ideas, recent snags suggest that getting gene editing into the medical mainstream isn’t going to be easy. This year saw the launch of the first clinical trials of CRISPR-based gene therapy in the US and Europe, using the technique to repair the faulty genes in blood stem cells that are responsible for causing sickle cell anaemia and beta-thalassaemia. Meanwhile, doctors in China are already recruiting patients into clinical trials testing CRISPR-modified immune cells that have been programmed to attack different types of cancer.

However, researchers at the Karolinska Institute in Sweden sounded a note of caution in June when they announced that CRISPR might increase the risk of cancer by activating an ‘immortalising’ response in cells. Other groups have raised fears of ‘off target’ effects – unintended accidental alterations in critical genes or control switches.
As a result, the FDA has put the current US CRISPR trial on hold, asking for more safety information. Ambiguous results from another gene editing study – this time using a related technique known as ‘zinc fingers’ to target a gene involved in a disease called Hunter syndrome – have also cast doubt on the effectiveness of the approach.
Patients and the public should therefore be cautious not to believe too much hype about gene editing over the coming years, warns Jackie Leach Scully, Professor of Social Ethics and Bioethics at Newcastle University. These treatments are only just entering clinical trials, and there’s a lot we still don’t know about their safety and effectiveness.
“I think we’re more sophisticated nowadays, more savvy and alert to the idea that these technologies get hyped up, but when you’re dealing with human disease and disability, there’s a great yearning among patients and families for something that works,” she says. “There’s a lot of hope around some of these technologies, but they could take decades to get here and may not be quite as good as we believed at the outset.”
Designing babies
The current CRISPR talk is mainly focused on editing cells in the body, known as somatic cells, creating changes that can’t be passed to future generations. However, Chinese scientists were the first in the world to announce that they had successfully managed to use gene editing to modify an early-stage human embryo back in 2015.
Since then, researchers in China, South Korea, the US and the UK have carried out similar experiments to fix various genetic disorders, although none of the modified embryos have ever been allowed to develop beyond a few days or implanted into a woman’s womb. Many researchers in the field are now arguing that it’s time to think seriously about how to move forward in this area, particularly for incurable, life-threatening conditions caused by single gene faults.
“I would say that in cases where there really is no alternative treatment, or preventative genetic screening, for a life-threatening condition then germline gene editing should be considered,” says Yalda Jamshidi, a genetics specialist at St George’s, University of London. “But this should only be done once the public and scientific and legislative communities have reached consensus about how we are going to use these techniques, how safe we need them to be, and whether we really are OK with it happening.”
China’s fast-paced research makes it likely the first germline edited baby will be born there, maybe even in the next decade
Although there is currently a ban on germline modification in most countries – altering genes in an embryo, eggs or sperm in such a way that could be passed on to future generations – it may only be a matter of time before this barrier is broken. Given China’s fast-paced research in this area, combined with the country’s willingness to reject international norms and dubious human rights record, it’s highly likely that the first germline edited baby will be born there, maybe even within the next decade.
So, there are a number of technical advances in gene editing that we can expect to happen over the coming few years: improving accuracy and reducing off-target edits; increasing the number of changes per genome; finding more efficient ways to deliver CRISPR into cells; and the adoption of new techniques such as precision base editing, which rearranges the atoms in individual ‘letters’ of DNA and RNA rather than cutting and pasting in new sequences.
While all these developments paint an exciting picture of the future, Leach Scully says that we need to think carefully about where else gene editing could lead us. “Most people talk about the use of gene editing to eradicate or at least reduce the impact of diseases and disabilities, but there is also the potential to augment or enhance current human capabilities through these technologies,” she says. “One is about making people better, the other is about making better people.”
Although most gene editing research is focused on healing the sick, there will be people out there working on ways to give healthy people an advantage, such as building bigger muscles, boosting brain power, improving sporting ability, or even creating a genetically ‘perfect’ human. The emergence of cheap and cheerful (or quick and dirty) ways of editing DNA to order has attracted the attention of groups that are keen to disrupt the established order of biotechnology research, from scrappy startups designing new organisms that produce useful drugs or break down toxic waste to infamous mavericks like ex-NASA scientist Josiah Zayner, who injected himself with muscle-enhancing CRISPR components during a live-streamed talk.
It may be a long way off, but having access to tools that can fundamentally alter our genomes raises big questions about what it means to be human and what we value in society.
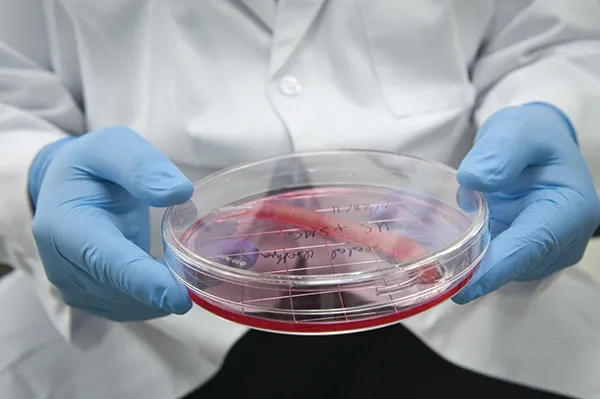
How CRISPR works
1
The CRISPR molecule is attached to ‘Cas9’ (shown here in beige).

This is an enzyme that uses its RNA ‘guide’ to recognise the target DNA sequence.
2
The CRISPR-Cas9 tool cuts the strands of the target DNA’s double helix...

Then the cell’s repair machinery will fix the damage – minus the old DNA sequence.
3
The CRISPR technique can be used to delete unwanted DNA, or to find and replace a sequence by adding genetic material – such as a new gene.

Spare parts
Stem cell technology has advanced to a point where scientists can grow replacement tissues or even whole organs in the lab. It’s also now possible to reprogramme cells from a small skin sample back into stem cells, then convert them into neurons, muscle, liver and more. Adding in gene editing makes for a powerful combination, fixing faulty genes in a patient’s own cells to make personalised ‘spare parts’. One idea coming down the pipeline includes using CRISPR to create ‘universal donor’ blood cells, which could be grown at industrial scale and given to patients needing blood transfusions or stem cell transplants. Scientists are also using gene editing to slice harmful viruses and incompatible genes out of pigs to make their organs suitable for human transplantation.
Listen to the programme Editing Life bbc.in/2F3CnEL
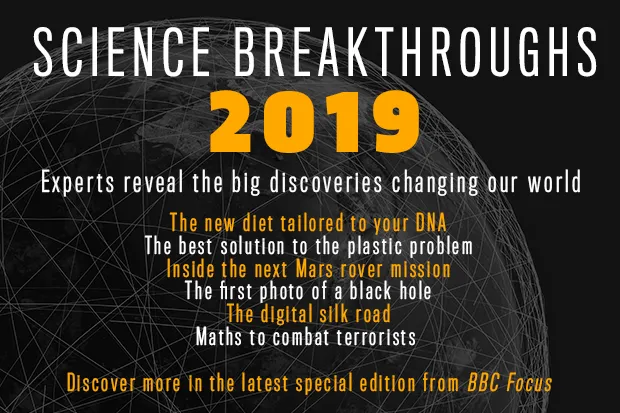
Follow Science Focus on Twitter, Facebook, Instagram and Flipboard