The greatest discovery in the history of science is that there was a day without a yesterday. The Universe has not existed forever. It was born. All matter, energy, space and even time, burst into being in a titanic fireball we call the Big Bang, 13.82 billion years ago. The fireball expanded and, out of the cooling debris, there congealed the galaxies – great islands of stars; two trillion of them, of which our Milky Way is but one.
Whatever way you look at it, the idea of the Universe popping into existence like a rabbit out of a hat is bonkers. For this reason, scientists had to be dragged kicking and screaming to it. The last thing they wanted to answer was the awkward question: what happened before the Big Bang?
In recent decades, the idea has taken hold that the Universe began with an ultra-brief burst of super-fast expansion. So violent was this ‘inflation’ that it’s been likened to the explosion of an H-bomb compared with the mere stick of dynamite of the more sedate Big Bang expansion that took over when inflation ran out of steam.
Read more:
- Where did the Big Bang take place?
- Why didn't the Big Bang produce equal amounts of matter and antimatter?
But now a prominent American astrophysicist is questioning the inflationary orthodoxy and advocating a fresh look at alternative models in which the Big Bang was actually a ‘big bounce’ from an earlier, contracting, phase of the Universe. “Crucially, my colleagues and I are proposing an observational test capable of distinguishing between the possibilities,” says Prof Abraham Loeb of the Harvard-Smithsonian Center for Astrophysics.
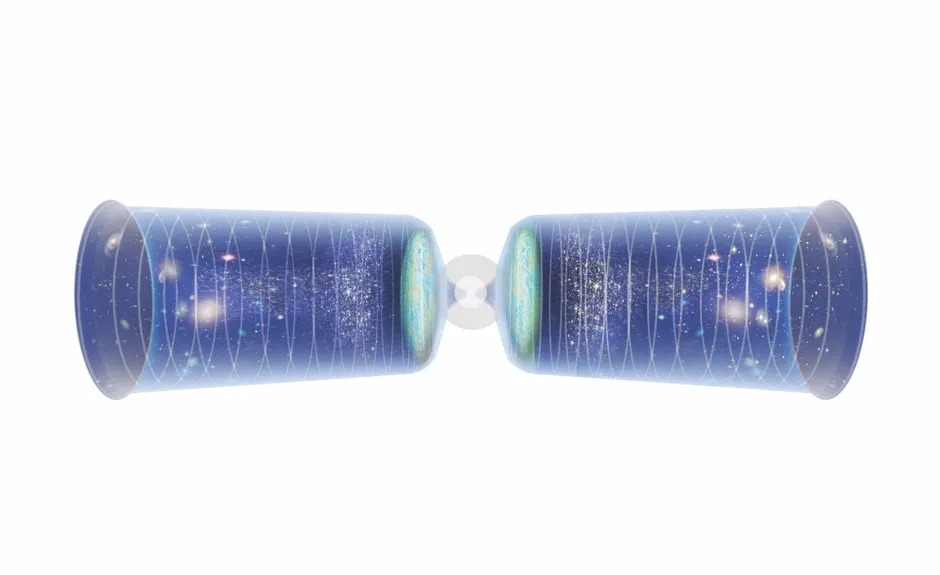
Inexplicably big gaps
One cosmic observation that any scenario must explain is why the Universe is so remarkably uniform: specifically, why the temperature of the heat afterglow of the Big Bang is pretty much the same everywhere and why the number of galaxies in a given volume is also the same everywhere.
This is a puzzle because if the expansion of the Universe is imagined running backwards to the Big Bang, like a movie playing in reverse, it becomes clear that regions of the Universe that today are widely separated were not in contact with each other at the beginning. In other words, there has been insufficient time since the birth of the Universe for any influence travelling at the cosmic speed limit (the speed of light) to pass between them. How, then, could heat have passed between such regions in order for their temperatures to equalise?
The standard explanation is that the Universe was far smaller early on than we imagine if we run that movie in reverse. If it was smaller, then bits of the Universe that are today widely separated would have been closer together. But, if the Universe started off smaller earlier on, it must have expanded faster in order to reach its current size in 13.82 billion years.
Such a period of super-fast expansion, occurring in the first split-second of the Universe’s existence, was proposed by the Russian physicist Alexei Starobinsky in 1979 and the American physicist Prof Alan Guth in 1980. This inflation was driven by the vacuum. Not the vacuum that we see around us today but a super-high-energy version. This was because the ‘inflationary vacuum’ contained a so-called scalar field, which, in common with the Higgs field, discovered at the Large Hadron Collider near Geneva in 2012, had a non-zero energy everywhere.
Read more:
- Dark energy is hiding in our Universe: here's how we'll find it
- Radical ideas: The number that holds the Universe together is changing
The super-high-energy inflationary vacuum had some remarkable properties. First, it had repulsive gravity, which caused it to expand – and the more of it there was, the greater was its repulsion and the faster it expanded. Second, when the inflationary vacuum doubled its volume, it doubled its energy; when it tripled its volume, it tripled its energy and so on. Imagine if you had a stack of banknotes between your hands and you pulled your hands apart and the result was the creation of more banknotes. Physicists, not surprisingly, refer to inflation as the ‘ultimate free lunch’.
But the inflationary vacuum, which contained energy but not matter, was a ‘quantum’ thing (quantum theory is our best description of the microscopic world of atoms and their constituents). And quantum things are fundamentally unpredictable. So, at random locations all over the inflationary vacuum, bits ‘decayed’ into normal, everyday vacuum.
Picture it as a vast ocean in which bubbles form. Inside each bubble, the enormous energy of the inflationary vacuum had to go somewhere. It went into creating matter and heating it to a ferociously high temperature – in short, into creating a big bang. We live inside one of these big bang bubbles in the inflationary vacuum.
Inflation decays at different rates in different locations, leading to 'bubbles' in the inflationary vacuum, in other words, creating the potential for a multiverse © Science Photo LibraryIn this picture, the Big Bang is not a one-off event. Big bangs are going off like fireworks across the inflationary vacuum. And all this could have been started by a small piece of inflationary vacuum – with a mass-energy of as little as a kilogram – popping into existence out of nothing, which, incredibly, is permitted by the laws of quantum theory. Inflation, once started, goes on forever since new vacuum is created faster than it’s eaten away.
But, according to Loeb, the inflationary scenario has problems. “After 40 years, we have no evidence of the existence of the ‘inflaton’ field that drives inflation, as we do of the Higgs,” he says. Also, there is an infinity of ways in which the inflaton field can decay, exiting inflationary expansion and starting Big Bang expansion.
Physicists imagine a mathematical ‘potential’, rather like a ski slope, down which the inflaton field slides, reducing its energy to zero. But the ski slope can have a different shape in different locations. “This means that inflation will continue for longer in some places than others, greatly changing nature of the resultant space-time,” says Loeb. “Inflation therefore predicts the existence of an infinity of domains, each with different physics – a ‘multiverse’.”

The problem is that our Universe doesn’t have the properties of a typical member of the multiverse. “Our universe is exceedingly dilute in vacuum energy relative to a typical region in the multiverse,” says Loeb.
We are therefore forced to explain its special properties, such as dark energy, with the topsy-turvy logic of the ‘anthropic principle’: that we live in the domain we live in because, if we didn’t, the physics would not have given rise to stars and galaxies, and physicists to describe them. “This gives inflation no explanatory power,” says Loeb. “It’s an infinitely flexible framework capable of fitting any data. To my mind that means it is not science.”
This claim, made by Loeb, Anna Ijjas and Prof Paul Steinhardt in a 2017 article in Scientific American, caused a storm of controversy. According to ‘the father of inflation’ Prof Alan Guth of the Massachusetts Institute of Technology: “The comment that inflationary cosmology, as we currently understand it, cannot be evaluated using the scientific method seemed so far removed from reality that 32 leaders in the field of cosmology, including five Nobel Laureates, and I wrote a letter to the editor categorically disagreeing with the statements made by this article about the testability of inflation.”

“That’s quite depressing,” says Loeb. “If a scientific idea is wrong, it needs only one person to point out why it’s wrong. Scientific truth is not decided by authority but by nature itself.”
Loeb thinks that inflation, like ‘string theory’ (a candidate for a theory of everything) has evolved over a long time in the absence of serious experimental tests of its fundamental ideas. “It has therefore created a culture in which supporters believe the theory need not pass the same stringent tests as other theories to prove its rightness,” he says.
Loeb does not necessarily think that inflation is wrong. But he thinks alternatives should be taken more seriously. “I have no disagreement with that,” says Guth. “But we should avoid making false claims that inflation can’t be evaluated by the scientific method. And I would also disagree with claims that any of the current alternatives to inflation have a comparable stature.”
From bang to bounce
One alternative is that the Universe didn’t begin with a burst of vacuum-driven inflation but instead underwent a previous contraction phase. The Big Bang would therefore not have been a Big Bang but a Big Bounce. There are a number of possibilities, one of which is the ‘cyclic Universe’ posited by physicists Steinhardt and Neil Turok, in which the Universe undergoes repeated bounces, possibly an infinite number of them, and therefore has no beginning.
Crucially, though, a long pre-Big Bang phase provides plenty of time for properties of the Universe to equalise, just as a long time allows a bath of cold water to come to an even temperature after hot water is added.
The key thing is to find an observation capable of distinguishing between inflationary and bouncing scenarios. And Loeb and his Harvard colleagues, Dr Xingang Chen and Zhong-Zhi Xianyu, say they’ve found one. It involves the heat afterglow of the Big Bang – the cosmic background radiation.
Read more:
- Did a tiny star power one of the biggest bangs in the Universe?
- Scientists create strange matter that once filled Universe
The Standard Model of particle physics describes the fundamental ‘fields’ that make up our Universe. An electron is a ripple in the ‘electron field’, a photon a ripple in the ‘electromagnetic field’ and so on. The Standard Model, however, is only an approximation of an as-yet-unknown deeper theory.
But Loeb says it will contain new fields with new massive subatomic particles. These will oscillate in the early Universe, imprinting a telltale regularity on the temperature variations of the cosmic background radiation. “This ‘periodicity’ is potentially observable,” says Loeb. “And the crucial thing is that it’s different for a Universe that underwent an inflationary expansion and one that underwent a contraction.”
The largest temperature variations are set in place first in an expanding scenario but last in a contracting scenario. And since the size, or amplitude, of the temperature variations grows with time, it is possible to tell which scenario was first.
“I’m glad to see that two years after proclaiming that inflation can’t be evaluated using the scientific method, Loeb is working on the development of a test of inflation,” says Guth. “The proposal by Chen, Loeb and Xianyu, in my opinion, contains some very interesting physics.”
The new test is not the only possible test of inflation. An experiment at the South Pole called BICEP2 is currently looking for the imprint on the cosmic background radiation of ripples in space-time (gravitational waves), created in the violent turmoil of the early Universe.

“If the imprint is found, it will prove inflation,” says Loeb. “But if the imprint is not found, it will be possible to find an inflationary model where the imprint is undetectable. This is what I mean about the theory being infinitely flexible and scientifically unfalsifiable.”
Loeb admits that the detailed physics of a cosmic bounce is as unknown as the detailed physics of inflation. But he says this is inevitable because of the state of our knowledge of fundamental science. The two towering achievements of 20th-Century physics are quantum theory (which describes the world of the very small) and Einstein’s theory of gravity (which describes the very large domain of the Universe).
In the Big Bang, a very big Universe was very small and therefore it is necessary to unify quantum theory and Einstein’s theory of gravity in order to predict what went on. Such a unification has so far proved elusive. “The stark truth”, says Loeb, “is that, without a quantum theory of gravity, we can never really be sure how our Universe began.”
- This article was first published in BBC Science Focus in April 2019 – subscribe here
Follow Science Focus onTwitter,Facebook, Instagramand Flipboard