Quantum physics describes the workings of the Universe at the level of the tiny particles, such as electrons and photons of light, that make it up. Most of our everyday experiences come from the interaction of matter particles with each other and with light, which can be explained by quantum electrodynamics (QED).
This detailed theory would win the Nobel Prize in Physics for Richard Feynman, Julian Schwinger, and Sin-Itiro Tomonaga. Much of their original thinking involved complex mathematics, as it was necessary to deal with a vast number of potential interactions, each with different probabilities.
But Feynman realised that these interactions could be represented by simple diagrams – patterns that define the interplay of light and matter. Not only did they make QED more comprehensible, Feynman diagrams also provided a visual tool for making otherwise impossible calculations practical.
Each diagram combines a series of lines where, for example, straight lines represent matter particles and wavy lines are photons. These are patterns in space-time, but they represent the interaction of particles. A simple example might show two electrons repelling each other electromagnetically, with a photon passing between them as a force carrier.
Feynman’s diagrams were necessary to reflect the strange behaviour of quantum particles, which bears no resemblance to the action of the physical objects that are made up of them. To see why the diagrams were so significant, we need to take a step back to what makes quantum physics appear so bizarre.
Read more about quantum physics:
- Quantum field theory: "An unholy crossbreed between quantum physics in a bad mood and every button you never push on a calculator"
- The bizarre link between bird migration and quantum physics
- How a quantum computer is different from your laptop, explained by a scientist
QED: Quantum electrodynamics
Quantum physics began with Einstein’s realisation that photons were real, soon expanding to explain the structure of the atom. It is the science of the very small, where reality seems not to have the deterministic certainty of the world we typically observe, but rather probabilities dominate.
Quantum electrodynamics, which is where Feynman diagrams were first used, considers the interactions of quantum particles that are dependent on electromagnetism. In common usage, ‘electromagnetism’ sounds like it is just about electricity and magnetism, which in a sense it is. But we need to understand that it is responsible for the vast bulk of everyday interactions in the world that we experience.
Light is an electromagnetic phenomenon. Similarly, most interactions between atoms is electromagnetic. So, for example, when you sit on a chair it is the electromagnetic force acting between the atoms of the chair and the atoms in your body that prevent your atoms from simply slipping past those in the chair.
The electromagnetic force is one of the four fundamental forces of the Universe. The other three are gravity, and the strong and weak interactions – these two forces are involved in the nucleus of atoms. We tend to think of gravity as pretty overwhelming, yet in fact it is by far the weakest of the four forces, billions of billions times weaker than electromagnetism.
If you doubt this, just think of a fridge magnet. The entire gravitational pull of Earth is attempting to pull it to the floor. All that holds it to the fridge is the electromagnetic force from its tiny magnet. The magnet wins.
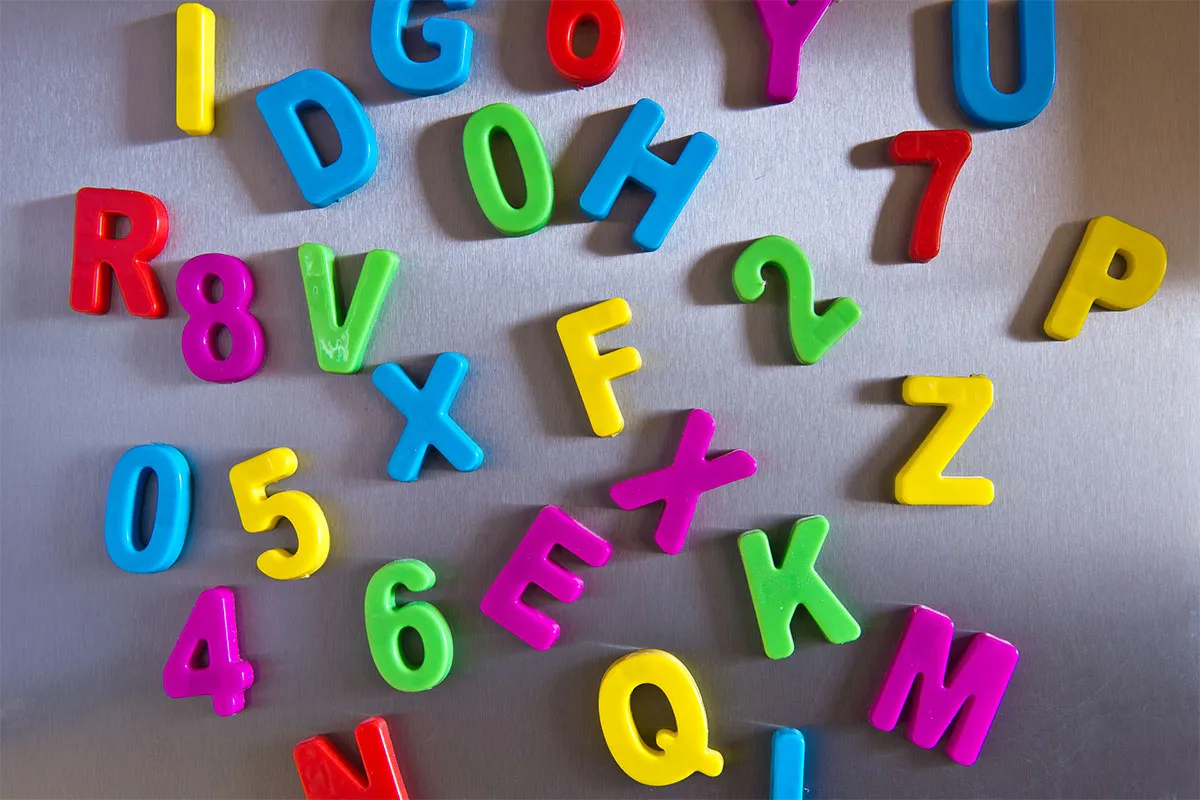
In quantum theory, forces pass from place to place as a result of so-called force carriers – particles that travel between the two objects that are attracting or repelling each other. This is why, for example, a magnet can attract a piece of iron at a distance. Perhaps surprisingly, the force carrier of electromagnetism is a particle we have already met – the photon.
We usually get introduced to the photon as the particle of light, but every time there is an electromagnetic interaction between matter particles a flow of ‘virtual photons’ between the particles produce the effect of a force. The term ‘virtual’ here is decidedly misleading. It sounds as if it means that the particles don’t exist. What it really refers to, though, is that the photons aren’t ever observed, as they pass from one particle to another without escaping.
As a result, nearly every electromagnetic interaction – which means nearly every interaction of matter not involving gravity – is the result of a matter particle emitting a photon, or a matter particle absorbing a photon, or both.
Feynman diagrams

Feynman diagrams are designed as a way both to illustrate those electromagnetic interactions, and to explore and quantify the many variants that the strange nature of quantum physics provides that would not be expected otherwise.
On the diagrams, matter particles are represented by straight lines and photons as wiggly lines. (There are other types of line when the use of the diagram is expanded beyond simple QED.) There is not a clear convention on which axis is time and space. Quite often, time is the vertical axis in a Feynman, but where it’s more convenient, it can be the horizontal axis instead.
The most common elements shown on such a diagram are that a photon travels from one location to another; a matter particle (at its simplest, an electron) travels from one place to another; or a matter particle gives off or absorbs a photon. Almost everything can be built up from these simple components.
But because of the oddities of quantum physics, an apparently simple action can result in a whole plethora of diagrams. Take an apparently simple example of two electrons moving. We know where they start and where they finish.
But how do they get from being located at A and B to being at C and D? The simplest possibility is the electron from A ends up at C, and that from B at D. Another probability is that the electron from A ends up at D and the one from B at C.

Note that we can’t tell which has happened as we don’t know the route the electrons took, nor do we know which electron is which. One of the definitive things about quantum particles, such as electrons, is that they have no distinguishing features. They are truly identical.
That’s straightforward enough, but there are other possibilities. Electrons, like other quantum particles, can undergo a process known as scattering. This is often represented as one electron bouncing off the other, like a pair of balls on a snooker table. However, electrons are electrically charged particles, and electromagnetic interaction works through photons.
So, another diagram would show a photon passing from one electron to the other, as a result changing the paths of the electrons so that they end up at C and D. This could arise in a number of ways.

Each of the different possible diagrams will have a probability attached to it. As we add in more unlikely possibilities, the outcome becomes closer and closer to reality. It’s amusing that quantum physics is in one sense the most accurate science we have. As Feynman once observed, the difference between its predictions and reality are comparable to the width of a hair on the scale of the distance between New York and Los Angeles.
Yet on the other hand, the predictions of quantum physics are based on probabilities, and though we can come nearer to the actual value it will always be the limit of taking every possible diagram into consideration, rather than a simple outcome.
Although Feynman diagrams sometimes have arrows indicating a direction of travel, they are often not necessary. Take, for example, the photon travelling between electrons in the diagrams shown above.
It is perfectly acceptable to say that the photon travels in the direction that has it travelling into the future, but in practice, the mathematics used to make the calculation doesn’t care whether the photon travels forward or backward in time. Just as the diagrams don’t usually distinguish a direction, so the photon is described as being “exchanged” by the particles, rather than travelling from a specific particle to the other one.
We won’t go through every possible diagram for this simple interaction (in fact it would be impossible, and even attempting it would be highly tedious). But just to show how more and more complexity could be added in, a next possibility could be to have two photons exchanged along the way, producing a pair of scattering events. By the time we get down to an event like this, the contribution is already something like one part in 10,000.
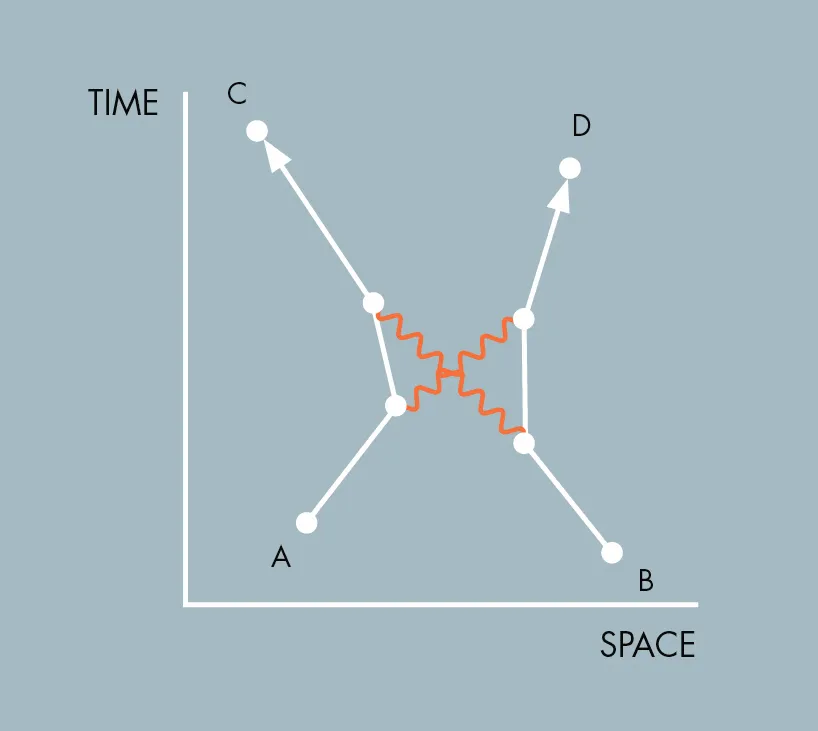
Note, incidentally, that the diagrams are more than visual illustrations – they are a mechanism to base calculations on. The actual calculations can become painfully messy, but the diagrams provide the patterns to ground them in an approachable fashion.
Ten Patterns That Explain The Universe by Brian Clegg is out now (£25, MIT Press).
- Buy now from Amazon UK, Waterstones or Bookshop.org
Read more about particle physics: