Long ago in the year AD132 the imperial astronomer Zhang Heng designed an earthquake detector. The History of the Later Han Dynasty reports that his ingenious invention would alert the Chinese emperor to catastrophic seismic events in remote outposts of the empire.
Zhang Heng’s seismoscope is described as a bronze vessel two metres in diameter with eight dragon heads mounted around its circumference. A reconstruction is shown below. Each dragon clasps a small metal ball in its teeth, while the open mouth of a bronze toad gapes wide below. A faint tremor from a distant earthquake causes a rod within the vessel to overbalance pulling a lever that opens the mouth of the dragon facing towards the earthquake. Its ball is released and falls clanging into the waiting mouth of a toad.
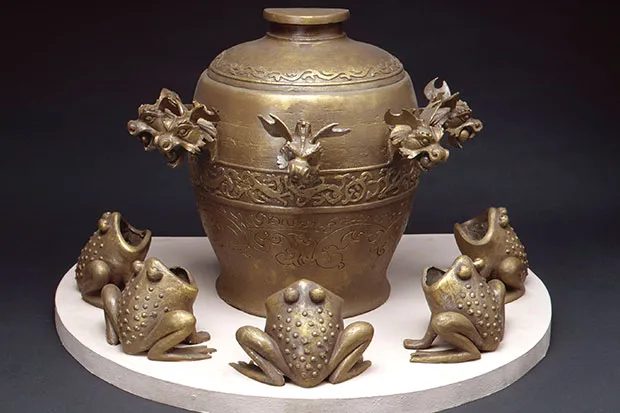
From Earthquakes to Spacequakes
Fast forward almost two thousand years and gravitational wave observatories now routinely detect spacequakes in far-flung regions of the Universe. In August 2017 the two LIGO detectors in the United States were joined by the newly upgraded VIRGO detector near Pisa in Italy. By determining the arrival time of faint cosmic rumbles at each detector with split second accuracy it is possible to get a good fix on the direction of the source of the gravitational ripples. This is valuable information as it enables astronomers to seek any visible sign of the catastrophic blast that produced the gravitational waves.
On 17 August the three instruments captured an unmistakable signal, quite different to the four previous events detected by LIGO. It was catalogued as GW170817. Just 1.7 seconds later NASA’s Fermi Gamma-Ray Space Telescope detected a short gamma ray burst emanating from the same region of sky and telescopes around the world were marshalled to locate the glowing embers of the event that produced the gamma radiation. Within twelve hours the source of the gamma ray burst was tracked down.

This was the first discovery of an optical counterpart for a gravitational wave signal and it has become one of the most observed events in the history of astronomy. The gravitational waves and short gamma ray burst were generated by a kilonova 138 million light years away in a galaxy known as NGC 4993.
Kilonovae form a new class of stellar explosions intermediate between novae and supernovae. They are around one thousand times brighter than a nova and this is the reason for their name. Nonetheless, they are just one-thousandth to one-hundredth of the luminosity of a supernova. We now know a kilonova is the spectacular explosion produced when a pair of neutron stars collide and merge.
Neutron Star Collisions
Neutron stars are like gigantic atomic nuclei—the entire mass of a star is compressed to nuclear densities. They are among the strangest objects in the cosmos. Typically, one and a half solar masses is packed into a sphere just 20 or 30 kilometres in diameter. Tiny by cosmic standards, but incredibly dense, the mass of a teaspoonful of neutron star far exceeds a billion tonnes. Try stirring that into your cup of tea!
The neutron stars whose collision generated the GW170817 event are presumed to have had a similar history to the Hulse–Taylor binary system explored in the previous chapter. Over the course of millions of years they would have gradually spiralled together as they lost energy due to the emission of gravitational waves. The amplitude of these waves was too small to detect until they came almost within touching distance 100 seconds before their fatal encounter.

Neutron stars are composed of an exotic form of matter consisting largely of neutrons. Such material only exists within the extreme gravitational stranglehold of a neutron star. Any fragment smashed free in a collision would be extremely unstable and undergo immediate radioactive decay with dramatic and violent consequences. Neutrons would transform into protons with the emission of electrons and neutrinos. Neutron-rich heavy nuclei would form and rapidly decay into more stable lighter nuclei in a blaze of gamma radiation.
This is the origin of the short gamma ray burst detected by the Fermi satellite. The ongoing radioactive decay of the material emitted in the merger produces the visible afterglow of the kilonova. The neutron stars that merged in the GW170817 event are believed to have undergone the ultimate collapse and formed a black hole.
The Birth of Heavy Metal
Neutron star collisions might answer one of the mysteries of the cosmos. How were heavyweight atoms such as platinum and gold created? Massive stars generate energy through a sequence of nuclear fusion processes that ultimately transform the core of the star into iron and nickel. Eventually, no further energy can be generated by fusion reactions and the core collapses, triggering the detonation of the star as a supernova. The exploding core of iron and nickel is bathed in a vast flux of high energy protons and neutrons and this was long thought to explain the origin of heavier nuclei all the way up to uranium and plutonium. However, recent computer simulations suggest the extreme conditions necessary for nucleosynthesis may not last long enough for the creation of elements beyond silver and its neighbours in the Periodic Table. This presents us with a conundrum: Where do all the really heavy elements come from?
David Eichler, Mario Livio, Tsvi Piran and David Schramms suggested in 1989 that neutron star collisions might provide an alternative process in which heavy elements are synthesized. This attracted little support at the time as it was assumed that such events would be too rare to account for the quantities of gold, uranium and other elements we find in the galaxy.
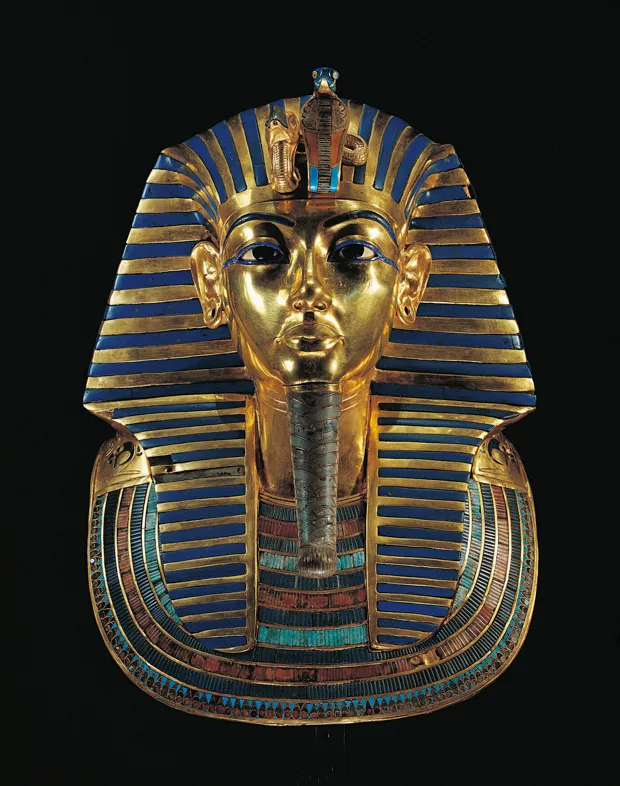
Interstellar Gold Rush
Computer models of neutron star mergers, such as the one that produced the GW170817 signal, show that around 20,000 times the mass of the Earth could be ejected in these events. This material would be blasted out at about one-fifth of the speed of light and dispersed far and wide throughout the galaxy. It would be in the form of heavy elements including about ten parts per million of gold nuclei, so the total amount of gold dust created in a kilonova would be about one-fifth of the mass of the Earth. Observations of the recent kilonova suggest that the computer models are correct.
But are these events frequent enough to account for the observed amounts of heavy elements?
Tightening the Net
The big question is whether heavy elements are produced in relatively small quantities in unusual events—supernovae—or whether they are produced in extremely large quantities in very rare events—neutron star collisions.
Support for the latter possibility comes from a recently discovered dwarf galaxy known as Reticulum II. Discovered in 2015 and located in the obscure southern constellation of Reticulum or the Little Net, this galaxy is a close companion of the Milky Way, just 97,000 light years away. Most dwarf galaxies contain little, if any, heavy elements. By contrast, significant amounts of the heavy elements are present in the stars and interstellar gas of Reticulum II.
Supernova explosions are unusual events. It is estimated that one occurs in the Milky Way Galaxy every 30 years or so, but most are hidden from us by interstellar dust clouds. Even in the feeblest of dwarf galaxies, we would expect a supernova at least once every 100,000 years, so if the heavy elements are produced in supernovae, over the 13.8-billion-year history of the universe the heavy elements would build up to significant levels. Neutron star collisions could be so rare, however, that most dwarf galaxies have never hosted such an event and this could explain why most dwarf galaxies do not contain heavy elements.
It looks as though the Reticulum II dwarf galaxy is simply the winner of the neutron star collision lottery. All the heavy elements that it contains were probably produced in a single such event. This provides important circumstantial evidence that long ago most of the heavy elements in our environment were also produced in this way.
The chart (above) indicates the origin of each element in the Periodic Table according to modern astrophysics. (The chart only shows the final process in which a particular nucleus is forged. Most nuclei are created following a sequence of possibly very different processes. For instance, boron nuclei are created when cosmic rays hit carbon nuclei and dislodge a proton, but the carbon nucleus would have been created in a star or supernova explosion.)
Your Gold Ring
It is an amazing fact that all the atoms in your body, other than the hydrogen atoms, must have been created within a star. It is perhaps even more incredible that the atoms in the gold ring on your finger were created in a neutron star collision. And what of the long gamma ray bursts? That is a topic for the next chapter.
This is an edited extract from The Cosmic Mystery Tour by Nicholas Mee, which is out 31 January 2019 (£16.99, OUP)
Follow Science Focus onTwitter,Facebook, Instagramand Flipboard