Stacks of little plastic dishes in a laboratory incubator, each one holding a free-floating blob of human brain might sound like the stuff of science fiction. But this is no futuristic flight of the imagination: these strange creations, known as brain organoids, are already being cultivated in labs all over the world, and researchers believe they could unlock some of the deepest secrets of how our brains grow and what happens when they go wrong.
“I don’t think that any of us set out to try and grow a brain in a dish,” says Madeline Lancaster, a neurobiologist at the MRC Laboratory of Molecular Biology in Cambridge. “If you’d asked me even just a few months before I started working on it, I would have said it was completely nuts – but in my case, it was an accident!”
Lancaster’s accidental experiments with organoids started when she was a postdoctoral researcher working in Vienna with molecular biologist Jürgen Knoblich, investigating how the brain forms during development in the womb. She started by growing brain stem cells in flat layers in a dish, but soon realised they lacked many of the key characteristics of nerve cells in a real brain. In search of a better method she tried a new technique for growing neural ‘rosettes’ – flat, flower-like circles of cells that were more realistic, albeit still two-dimensional.
Read more:
“When I put the cells in the culture dish, there was something wrong with the reagents that I was using,” she says. “Rather than forming these nice flat rosettes, mine were forming these weird, floating balls. I thought they looked interesting, so I continued growing them.”
Speaking to other researchers in the field, she discovered that some of them had also seen these strange blobs, but had thrown them away because they looked wrong. But while these brain balls looked curious from the outside, what Lancaster found inside was fascinating. Each was made from bulging layers of cells connected by cavities, just like the fluid-filled ventricles that connect the hemispheres of the cerebral cortex in a real brain. Even the layers of cells mimicked the arrangement in normal brain tissue, with stem cells lining the ventricles and layers upon layers of more specialised cells and neurons built up towards the outside.
Building a brain
Despite their ‘mini-brain’ nickname, these organoids are a long way from being full-size human organs. They’re around half a centimetre in diameter – roughly the shape and size of the eraser on the end of a pencil – and they lack key structures such as blood vessels, which limits how big they can grow. Organoids are also remarkably hardy, as long as they’re grown in a scrupulously clean environment, and can stay alive for more than a year.
Lancaster’s mini-brains are enabling her to prise open the ‘black box’ of human brain development. Because they reflect the cell types and organisation of a growing human brain, organoids are opening a window into time of life that has previously been inaccessible to science.

“People have done MRIscanning on children and even babies to and look how the brain wiring changes, but when it comes to those early events – how neurons are made, how many, which types and where – we can’t answer them, no matter how good our MRI machine is. But I think what’s happening in these dishes reflects what’s happening in an actual embryo. We know this because the end product looks like a lot like a real brain, so we have a tractable system to start asking some of these fundamental questions about brain development.”
Lancaster is also using her mini-brains to answer an even deeper question: what makes a human brain human? We share more than 95 per cent of our DNA with our closest primate relatives, such as chimpanzees, but our brains are much bigger and undoubtedly different. By comparing brain organoids grown from chimp stem cells with those from humans, she and her team are watching how these differences emerge from the earliest stages of development. There’s even the possibility of using new genetic engineering techniques to switch human and chimp genes around in mini-brains – something that would be impossible to do in living animals – to pin down the precise molecular pathways that make the human brain so special.
The brain-like appearance of these organoids raises ethical as well as scientific questions. Can they think, and are they conscious? According to Lancaster, the answer is almost certainly no.
“I think of them as being a bit like brain tumours,” she says. “Tumours contain many more neurons than our mini-brains in a dish, but no one is concerned that their brain tumour is thinking or has consciousness, and nobody is sad that it has been taken out and thrown away. That’s what we have here. It’s not an organised network, and it cannot make a functional thinking circuit – it’s a ball of brain tissue, and just because you have neurons doesn’t mean it can think.”
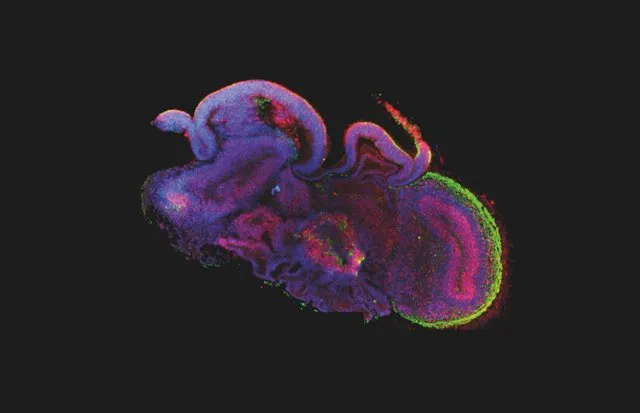
Today, she and her team are growing mini-brains from human embryonic stem cell lines – the multi-purpose cells originally found in very early human embryos, but now cultivated in the lab. She’s also using so-called induced pluripotent stem (IPS) cells: adult cells that have been pushed back to an embryonic state with a cocktail of molecules first discovered by Nobel Prize-winning Japanese scientist Shinya Yamanaka. Depending on the exact conditions used, Lancaster can nudge her organoids to develop all kinds of cells, from the fluffy choroid plexus (which would connect with blood vessels in a real brain) to pigmented light-sensing cells that are usually found in the retina at the back of the eye.
“There’s just so many cell types to look for,” she says, “But depending on the method we use, every time we look for something that we know should be there, we find it.”
Wiring up
Mini-brains don’t just allow researchers to study normal developmental processes. Sergiu Pasca, Assistant Professor of Psychiatry and Behavioural Sciences at Stanford University in California, is using them to understand what goes wrong in autism, schizophrenia, epilepsy and other neuropsychiatric disorders.
“Most of the psychiatric drugs we have today have been discovered by chance – we know very little about the origins of these disorders and the question is why?,” he asks. “Unlike cancer biologists, who can take out a tumour, put it in a dish and find ways to treat it, we cannot do that with the brains of our patients with mental disorders.”
Pasca and his team have managed to grow mini-brains for more than two years – a staggering 800 days is their current record – and shown that they can generate most of the same cell types and structures found in real human brains. They’re using the technique to investigate the roots of severe autism and epilepsy syndromes, by generating organoids with IPS cells derived from skin samples of affected children and then carefully comparing them with cultures grown from healthy cells.
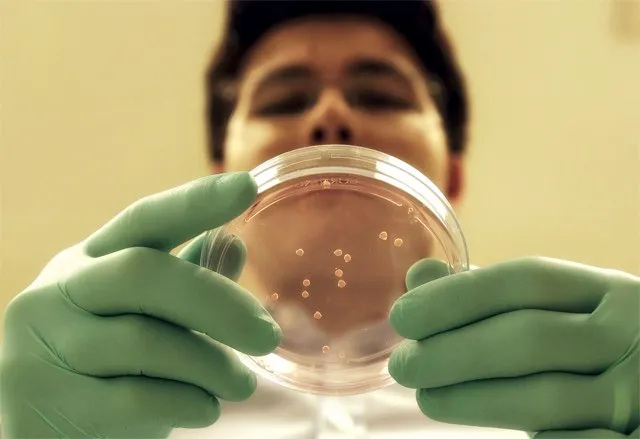
“We can use electrodes to measure how the cells are talking to each other, and microscopy to see how the cells move and make connections with each other,” he explains. “Many of the genes associated with these disorders are involved in the connections between nerve cells, so we can see how the gene changes in these patients are impairing the communication within the brain in a non-invasive way.”
He’s now taking these ideas even further, sticking together organoids that mimic different regions of the brain and studying their interactions – a technique he describes as ‘brain Lego’. The team is using these hybrids to spy on the brain as it wires itself up, focusing on what happens to so-called inhibitory neurons that normally help to calm down brain activity but are faulty in people with epilepsy and autism.
“Inhibitory neurons are not born in the cortex on the surface of the brain: they are born in a very deep region of the forebrain and have to migrate millimetres over many months after birth,” Pasca says. “It’s really fascinating to watch in our cultures – they kind of pull themselves up and jump along.”
But when Pasca and his colleagues looked at organoids grown using cells from patients with a form of autism that is associated with epilepsy, they saw a very different picture. The inhibitory cells were moving in a very peculiar way, jumping more often but less efficiently and eventually getting left behind.
Impressively, the researchers were then able to identify a drug that could rescue these lagging cells, correcting the wiring defect and pointing towards a potential future treatment for children suffering from the same condition.
Into old age
Meanwhile at University College London, neurologist Selina Wray is using brain organoids to look at neurodegenerative conditions that start at the other end of life, including Alzheimer’s disease and fronto-temporal dementia.
“Normally we have to work with post-mortem brain tissue from patients, but you’re only ever looking at the end stages,” she says. “It’s almost like coming to the scene of a crime after the criminal is gone, and you’re trying to piece together a sequence of events by looking at the damage that’s been left. I want to build models in the lab which will let us look at the very beginning of the disease – because if we understand the first things to go wrong, that’s when treatment should be more effective.”
Read more:
- Should medicine be gendered?
- Why personalised medicine is coming, and how it’s going to help us beat disease
In a similar way to Pasca and Lancaster, she’s taking samples of skin from patients with dementia, turning them into IPS cells and then growing organoids. Wray can spot differences compared with organoids from unaffected people after just a few months, finding increased levels of the forms of certain molecules that are associated with Alzheimer’s disease.
However, there’s a problem with this approach: mini-brains mimic the very earliest stages of life, while dementia is a problem that takes decades to develop. To solve this, researchers are working on clever hacks to speed up the ageing process. One idea is to add in genetic changes that mimic progeria – a rare disorder that causes dramatic premature ageing. Another approach is to meddle with the structures protecting the ends of DNA inside cells, known as telomeres, which act as a kind of countdown clock as we age.
As well as studying the underlying processes that drive dementia, Wray thinks that mini-brains have a lot of potential for helping to identify the right treatment for individual patients.
“I feel excited by the idea of personalised medicine – that you could take somebody’s cells and grow organoids in the lab, screen a panel of drugs against them and say, ‘Okay, we think this person will respond to drugs ABC, but this person will respond better to drugs XYZ,’” she says. “That’s happening in cancer biology, this idea of being able to stratify patients on a molecular basis, and while I think we are a long way off, I love the idea of growing someone’s neurons so we can work out what therapies we should be giving them.”
Sergiu Pasca is similarly enthusiastic about the potential of mini-brains to change lives.
“Our organoids are grown from cells taken from real patients,” he says. “These kids have severe neurodevelopmental disorders that really impair their lives, and to think that a few months later you can derive brain tissue from those patients in a dish and start asking questions about how the disease may arise – that’s what makes this exciting.”
- This article was first published in December 2017
Follow Science Focus onTwitter,Facebook, Instagramand Flipboard