Earlier this month, officials at CERN, the world-leading particle physics research organisation, took another step towards building what will be the world’s largest particle accelerator – a machine designed to smash molecules together at speeds close to the speed of light.
At a gigantic 91km in length, the proposed supercollider will be three times the size of the Large Hadron Collider (LHC). The new machine, dubbed the Future Circular Collider (FCC), will allow scientists to smash particles together with greater precision and higher energy than ever before.
It is, to put it mildly, a big deal. The hope is that the FCC will help scientists solve some of the biggest mysteries in the Universe, such as why there is more matter than antimatter, what dark matter and energy consist of, whether there are hidden extra dimensions and even how the entire Universe could end. In short, it could completely blow apart our knowledge of the most basic building blocks of our cosmos.
“Our current Standard Model of particle physics is thought to be an approximation of a deeper, more fundamental theory because it fails to explain why the particles have the masses they have; why forces have the strengths they have; and it makes no reference to the mysterious dark matter and dark energy, which make up 95 per cent of the mass-energy of the Universe,” says science author Marcus Chown.
“Unfortunately for physicists, the Standard Model is also stunningly good at predicting what is observed in experiments, forcing physicists to hunt for hints of the deeper theory in the nth decimal place of their measurements. The Future Circular Collider would be a powerful weapon in this hunt."
If the plans are approved by the CERN Council, construction of the colossal collider could begin by the mid-2030s, with the first phase operational around 2045. A planned second phase extends research into the 2070s, marking the FCC as a multi-generational scientific endeavour.
undefined
Is bigger always better?
When it comes to building particle accelerators, size matters. Part of the reason is that larger accelerators can achieve higher collision energies. And since energy is related to mass through Einstein’s famous equation, this allows you to make matter.
“When we look for new particles like the Higgs boson, you have to put in enough energy to create it,” explains Prof Andy Parker, a physicist at Cambridge University, who chairs the scientific advisory committee for the FCC. “Good old E = mc2 tells us how much energy we need to put in.”
Even though the masses of particles are tiny, they’re multiplied by the speed of light (that’s the 'c' in the equation) squared, which is a really big number – 299,792,458 metres per second, to be precise. But you can’t speed up particles to that speed instantly – they need to be accelerated over long distances to reach speeds close to the speed of light themselves.
“We know for a fact that there are holes in our current model of how particle physics and, in general, physics work. And we believe that the explanations for those lie in particles with higher masses than we have at the moment,” Parker continues.
“So, in order to explore what happens and how these solutions work, we have to look for particles at higher energies than we have seen so far.”
While the LHC is capable of achieving collision energies of 14 teraelectronvolts (TeV), the FCC will eventually aim to reach energies seven times greater in the search for a new and complete understanding of physics.
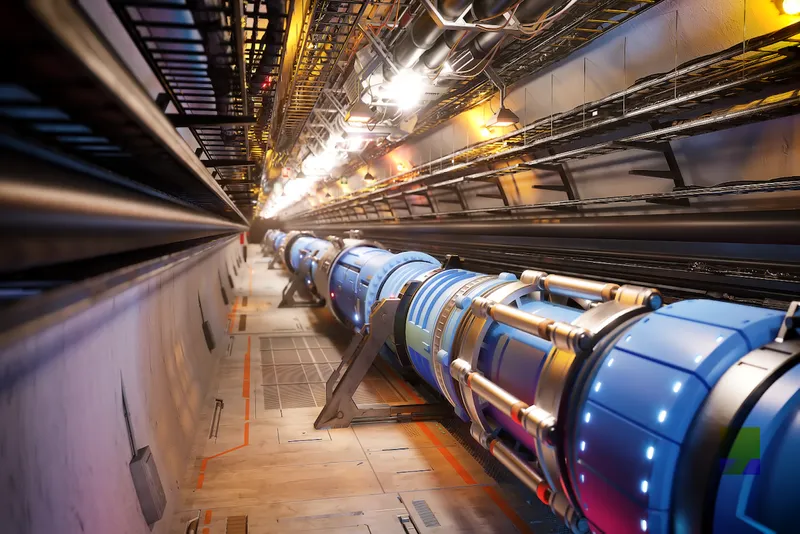
The god particle
Initially, the FCC will look to dramatically increase the precision of measurements physicists can make. It will collide electrons and their antimatter counterparts, or positrons, with the aim of producing millions of a specific particle: the Higgs boson.
“We call it a Higgs factory because it will produce millions of Higgs bosons,” says Matthew Mccullough, a theoretical physicist at CERN. “And because it's an electron-positron collider, it's a very clean environment, so you can study the properties of the Higgs boson with exquisite detail.”
The Higgs boson is a carrier particle of the Higgs field which permeates space and gives other particles their mass.
It might seem intuitive that matter has mass because, well, it’s made up of stuff – protons, neutrons and electrons, for example. However, the existence of the Higgs boson and field flips that notion on its head.
“What the Higgs boson says is that none of it has any substance,” says Parker. “It only comes from the interactions between things; the interaction with the Higgs field causes it to look like a solid particle.
“If I pick up my teacup, it only feels like a solid lump of teacup because of the Higgs field interacting with it.”
However, since the Higgs boson’s discovery using the LHC was announced on 4 July 2012, scientists have struggled to find out much more about it.
“We really don’t understand how the Higgs field really operates and how it compares to the rest of the particles that we see in the Universe,” Parker continues. “There's something missing there.”
According to McCullough, the first stage of the FCC will be like “taking a surgeon’s scalpel to the Higgs boson”, allowing scientists to figure out exactly what is going on inside it. “Is it made of something? Is it interacting with other particles that we can't yet produce?” he says.
Just like how atoms were once thought to be fundamental, then revealed to be intricate systems of smaller particles, McCullough thinks the same could be true of the Higgs boson.
“When we've discovered particles like that in the past, they have turned out to be composite. They've turned out to be one layer of reality,” he says.
Read more
- What has the discovery of the Higgs boson taught us?
- CERN: Everything you need to know
- How new antimatter science could soon explain the existence of everything
The beginning and the end of the Universe
While little is known about what could make up the Higgs boson and the mechanisms by which it interacts, scientists have a good idea of its importance in the Universe.
In the very early Universe, nanoseconds after the Big Bang, matter was so hot and dense that it moved at the speed of light and was completely massless; the Higgs field was zero. But as the Universe grew and cooled, the Higgs field is thought to have spontaneously begun interacting with matter, giving the Universe mass.
McCullough described this as a moment of transition, similar to steam condensing into water, where the Higgs boson 'switched on' and bestowed mass upon particles.
Similarly, the Higgs boson is important for understanding how the Universe might end since it affects the stability of the Universe itself.
According to current understanding, the Universe is expanding at an accelerating rate and will continue to do so until the eventual ‘heat death’ of everything, everywhere. But this scenario is by no means definite and hinges on our understanding of the Higgs boson.
“If you do the calculations with our current understanding of the Higgs Boson, we’re right on the boundary between instability and stability,” Parker says, whereby a stable Universe would continue expanding as things are, but an unstable one could collapse into a completely new state.
By uncovering the mysteries hidden within the Higgs boson using the FCC, scientists are hoping to finally answer the fundamental question: How does it all end?
To explain the Higgs boson’s effect on the fate of everything, Parker likens our Universe to a ball rolling down a hill. The ball will settle at a low, or stable, point, and energy will be required to move it again.
“Are we sitting in a Universe where everything has reached the bottom of the hill?” Parker asks. “In which case, you're not going to fall any further, and the Universe will sit where it is and just carry on expanding and getting colder forever.”
However, whether or not our Universe has settled at a local minimum point or the lowest possible state is dependent on the exact mass of the Higgs boson, something researchers hope to measure more accurately using the FCC.
“Or, could the Universe be in a higher energy state waiting to roll down another hill?” he continues. “Such a scenario could mean that the Universe could spontaneously collapse into a new state.”
If we do have another hill to roll down, this could be consistent with the idea of a cyclical Universe. In this model, the Universe wouldn't undergo perpetual expansion and eventual heat death, but rather a grand collapse and a potential ‘Big Crunch’, followed by a potential Big Bang, restarting the cosmic cycle.
“I think we would like to know whether we’re in a stable Universe or an unstable one. I really think that's a great question,” Parker concludes. The FCC could hold the key to answering it.
Read more
- CERN: Everything you need to know
- How the hunt for invisible particles is opening a new scientific frontier
- No, you absolutely cannot drop candy into the Large Hadron Collider. Here's why
About our experts
Marcus Chown is an award-winning writer and broadcaster and a former radio astronomer at the California Institute of Technology in Pasadena. He is the author of Breakthrough: Spectacular stories of scientific discovery from the Higgs particle to black holes (Faber & Faber, 2021).
Professor Andy Parker is a British physicist and professor of high-energy physics at the University of Cambridge. He is the incumbent Master of Peterhouse, Cambridge, as well as a Fellow of the Institute of Physics and an honorary Professor at the British-Kazakh University in Almaty. Previously, he was the project leader for one of the major detector systems used by the ATLAS Collaboration at the CERN Large Hadron Collider (LHC) for the discovery of the Higgs boson in 2012 and is now a member of the CERN Science Policy Committee and chair of the Scientific Advisory Committee for the Future Circular Collider.
Matthew McCullough is a theoretical physicist and researcher at CERN. His fields of interest include, beyond the standard model phenomenology, collider physics, cosmology and astroparticle physics and quantum field theory. He is involved in the FCC collaboration on the FCC feasibility study.