How do you spot an alien? Some scientists are looking for communication signals beamed out into space. Others propose looking for dips in starlight that might be caused by huge alien-built megastructures orbiting a distant sun.
But perhaps the most promising line of enquiry lies with probing the layer of gases surrounding alien worlds. If we were to watch Earth from afar, we would be able to infer our existence by analysing the make-up of our atmosphere. There’s only one process we know of that could keep it so rich in oxygen: life.
If aliens live on other worlds, they’d probably also imprint a signature of their existence in their atmospheres. While we are able to peer at alien atmospheres through the latest space telescopes, there’s a catch: we don’t really know what we’re looking for. We only have Earth as a comparison – but what if there are other combinations of gases that can reveal the presence of life?
Read more about the search for alien life:
The answer to this question may lie in intriguing new research that’s replicating alien air on Earth, cooking up exotic brews in laboratories. Meanwhile, other scientists are simulating the weather and circulation of alien atmospheres inside powerful supercomputers to find out just how hospitable distant worlds may be. This research is already providing some tantalising clues about where the next generation of alien hunters should be focusing their attention.
Something in the air
Since the first detection of an exoplanet – a planet around another star – in 1992, more than 4,000 have been identified, mostly by observing the subtle but regular dimming in starlight as the planet passes across its parent star and blocks some of its light (a transit). More than half of exoplanets were detected in this way by NASA’s Kepler space telescope (active from 2009 to October 2018). In April 2018, NASA launched a successor to Kepler: the Transiting Exoplanet Survey Satellite (TESS).

In order to study an exoplanet’s atmosphere, astronomers look at how the atmosphere absorbs the starlight passing through it. Different molecules of gas will absorb different wavelengths of light, so researchers can analyse the star’s filtered light spectrum during a transit to pin down which gases are present. In this way, astronomers made the first direct detection and chemical analysis of an exoplanet atmosphere in 2001 – finding sodium in the atmosphere of a gas giant known as HD 209458 b.
Since then, several exoplanets have had their atmospheres analysed, revealing the presence of water vapour, methane, carbon dioxide, and even small amounts of oxygen around some of these worlds. None of these gases alone signals life, however – not even oxygen, as we know of processes that can create small amounts of it without involving living organisms.
This is where the work of planetary scientist Dr Sarah Hörst comes in. At Johns Hopkins University in Baltimore, US, she is leading a team of scientists who are brewing lab simulations of the gases likely to be in exoplanet atmospheres, in order to find out what they might produce. So far, Hörst’s work has focused on an atmospheric phenomenon that’ll be familiar to anyone who’s spent time in a big city: haze.
Cooking with gas
The two most common types of exoplanet have no equivalent in our own Solar System. One is the ‘super-Earth’: rocky, with a diameter 1.25 to two times that of Earth. The other is the ‘mini-Neptune’: about two to four times the size of our planet, with a thick blanket of gases (mostly hydrogen and helium) over a dense core of rock or ice.
Astronomers have discovered that the atmospheres of super-Earths and mini-Neptunes are rather thick and misty: light doesn’t get through them easily. This could be because they’re full of clouds (perhaps made from droplets condensed from water vapour or other gases such as methane), or it might be due to haze: tiny, dust-like solid particles, like the pall over cities caused by traffic fumes.
Hörst is trying to find out. She says that there might be ramifications for whether planets like this could support life. According to Hörst, haze particles in an atmosphere can have a huge impact on how starlight moves through it. “This can affect things like how much and what sort of energy is available at the surface of a planet for life, and what the temperature of the surface is,” she says.
We have only the sketchiest information about the chemistry of these atmospheres, so Hörst carries out simulations for a wide range of possible compositions, including all the common gases likely to be found around worlds like these: water vapour, carbon monoxide, carbon dioxide, nitrogen, hydrogen, helium and methane. Hörst mixes up different proportions of these gases at temperatures between about 25°C and 325°C, mimicking the conditions thought to exist on super-Earths and mini-Neptunes.
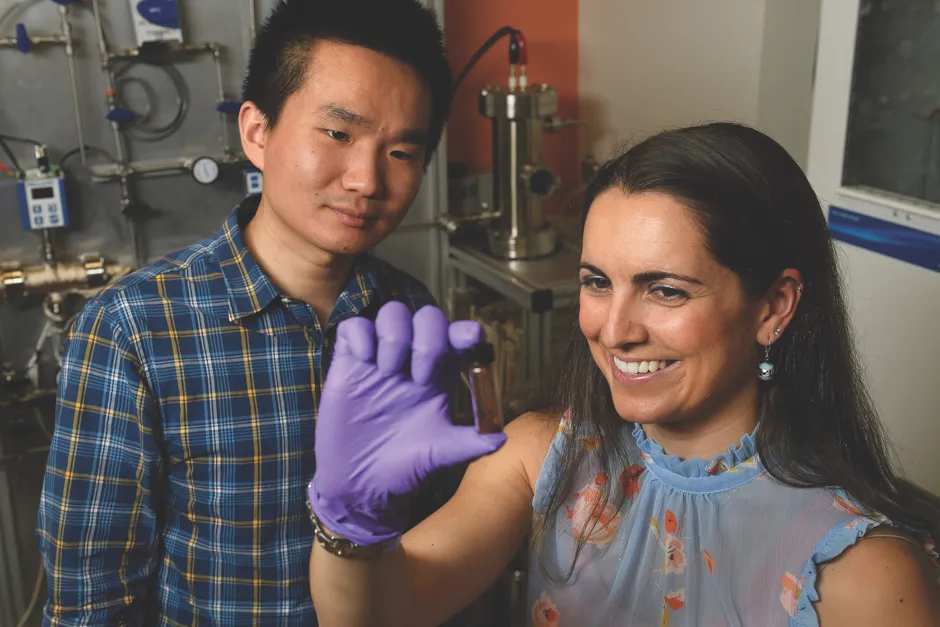
It’s a kind of cosmic cookery: throw together the ingredients, bake at moderate heat, and see what comes out. There’s another crucial ingredient, too: energy to kick off chemical reactions by breaking molecules apart. On exoplanets, this could come from high-energy ultraviolet rays in the starlight, or from electrically charged particles formed by cosmic rays flooding into the upper regions of the atmosphere. The researchers simulate these energy sources using either an ultraviolet lamp or an electrical discharge like that in a fluorescent lighting tube.
Most of the mixtures that Hörst and her team have studied generated brownish, smog-like haze, similar to what we see on Saturn’s moon Titan. The amount of haze varied widely, though, depending on the composition of the mixture. For example, two of the experiments with plenty of water vapour and methane produced the most haze, but a third experiment also generated fine particles with no methane present at all.
More work is needed to find out what the detection of haze on a distant exoplanet would mean for the likelihood of finding life. Hörst says that in some cases haze might block harmful radiation (as the ozone layer does on Earth), but it could also lead to cooling of the surface and a lack of liquid water. “We need to know more about the planet and its atmosphere to be able to understand what the conditions might be like on the surface, and what processes would have led to the formation of haze,” she says.
Read more about exoplanets:
In the meantime, the holy grail of this area of research is to identify some molecule – or a set of molecules – that can exist only if there is life, i.e. a ‘biosignature’ of alien life. But what might that be?
The answer doesn’t appear to be oxygen alone. Hörst and colleagues saw oxygen form in their simulation experiments, purely from chemical reactions induced by ultraviolet light. They also saw organic molecules like ethanol and formaldehyde, which rules these out as definite biosignatures, too.
One possible biosignature is the simultaneous existence of ozone and methane, says Hörst. This is a chemically unstable blend of gases, and there’s no known geological process that could sustain them. “It would be really hard to have them together in the same atmosphere without some source replenishing them,” says Hörst. On Earth, the biosphere is ultimately the source of both of these gases in our atmosphere.
Some of the most enticing worlds to look for biosignatures such as these, Hörst feels, are the set of planets detected in 2015 around a dim star called TRAPPIST-1, located 40 light-years away in the constellation of Aquarius. Seven of the worlds orbiting this star are vaguely Earth-like, and most of them are potentially habitable, having the right conditions for liquid water on their surfaces. By looking at the light transmitted through their atmospheres, Hörst and coworkers have found that some of them may have clouds or haze, though it’s hard to be more precise at this stage about which option is more likely.
Hörst thinks that these intriguing planets should be an early target for NASA’s James Webb Space Telescope (JWST), the planned successor to the Hubble Space Telescope, which will take a closer look at exoplanet atmospheres once it's been launched in 2021.

Exo-climates
Life on Earth relies not only on having the right kind of atmosphere, but also on the whole climate system: how air, oceans and heat circulate, and how clouds form. If we found an exoplanet with an identical atmospheric composition to Earth, it might still be inhospitable to life if it lacked a similar climate system. A new research area called ‘exoclimatology’ is aiming to understand exoplanet climates – and their implications for life – by applying the computer models used to simulate the Earth’s weather and climate to other worlds.
So far, much of the work has focused on another common exoplanet type: ‘hot Jupiters’ – gas giants like our own Jupiter but which orbit much closer to their parent star. They tend to either rotate very slowly or be ‘tidally locked’ so that – as with the Moon orbiting the Earth – the same side always faces the star. This gives the planets a temperature difference between the ‘day’ side and the ‘night’ side which drives atmospheric circulation, much as the temperature difference between Earth’s equator and the poles powers our own climate.
Read more about Earth's climate:
- The effects of the Little Ice Age went far beyond climate change
- February heatwave: is the UK climate changing?
Computer models of this circulation indicate that hot Jupiters have a kind of atmospheric jet stream, says Dr Nathan Mayne, who leads the exoclimatology group at the University of Exeter. This can mix up the chemistry of the hot and cool sides of the atmosphere, changing the blend of gases in some places and thus the amount of starlight that’s transmitted to the surface. While hot Jupiters are not likely to harbour life, this shows how the circulation of the atmosphere can play a crucial role in the surface conditions on a planet – with important implications for their habitability.
Add water to the equation and things get even more interesting. Some potentially habitable planets like the TRAPPIST-1 group are also likely to be tidally locked to their star. If these exoplanets have liquid water on their surface, then water on the hot day side will evaporate, eventually condensing into rain or snow on the cooler night side.
“Land covering the day side would quickly dry out and the moisture would be transported to the night side,” says Mayne. “But if there’s an ocean, the water can circulate back” – creating a giant conveyor belt of water between the two sides of the planet. This could make the difference between a barren planet divided into halves – each too extreme for life to exist – and a planet where water circulation creates a more moist and clement environment.
With the latest generation of space telescopes, it won’t be long before we’re able to study the atmospheres of ever more exotic exoplanets. “The combination of TESS and the JWST should provide us with a lot of compelling worlds to study,” says Hörst. Work by the likes of her and Mayne is set to become crucial to astronomers who want to know whether the exoplanet gases and weather patterns that they’re detecting are the symptoms of a sterile planet, or maybe – just maybe – hints of life…
- This feature was first published in the July issue of BBC Science Focus - subscribe here.
Follow Science Focus onTwitter,Facebook, Instagramand Flipboard