After the Sun, Earth’s Moon is the most obvious object in our skies – an ever-changing but reassuringly permanent presence in the heavens. Yet the origins of the Moon were a mystery for astronomers until recently, and may still not be entirely resolved.
Many ancient cultures from around the world had their own legends about the origin of the Moon. Most were linked to a mythological understanding of the cosmos that considered the Sun, Moon and stars as avatars of the gods.
As far as we know, the ancient Greeks were the first to treat the Moon as a physical object in space, but the idea of the heavens as a celestial clockwork, maintained by divine power throughout eternity, went largely unchallenged into late medieval times.
The first detailed theory to explain the origins of Earth and the Solar System in scientific terms was the ‘nebular hypothesis’ proposed by the Swedish philosopher Immanuel Swedenborg in 1735, and developed by the French mathematician Pierre-Simon Laplace in 1796. In this model, interstellar nebulae – clouds of gas and dust between the stars – collapse and coalesce to form the stars and planets that surround them.
Read more about the moon:
- Why is the Moon sometimes visible in the day?
- What if we mined the Moon?
- Race to the Moon: Inside China's plans to build a lunar base
- Why we need to go back to the Moon
The worlds of the Solar System therefore grew in stages from the bottom up, and collisions between them released enough heat to melt and separate them into layers of different densities. Laplace’s theory has since turned out to be substantially correct, although it spent much of the 19th and early 20th Centuries out of favour.
For Laplace, the most obvious explanation for the Moon was that it must have formed alongside Earth (a process known as binary accretion) and been a constant companion since the earliest days of our planet’s history.
But even Laplace could see that the Moon’s huge size presented a problem. With a diameter more than a quarter of Earth’s own, it is by far the largest satellite compared to its planet in the Solar System. This seemed to demand that Earth’s formation left behind an unfeasibly large amount of excess material.
Ironically, Laplace’s theory fell temporarily from grace – replaced by a cold-formation theory – before the problem of the Moon’s diameter could undermine it. This left the way open for other theories. Perhaps the most promising alternative was put forward by astronomer and mathematician George Darwin, son of the great naturalist Charles, in 1879.
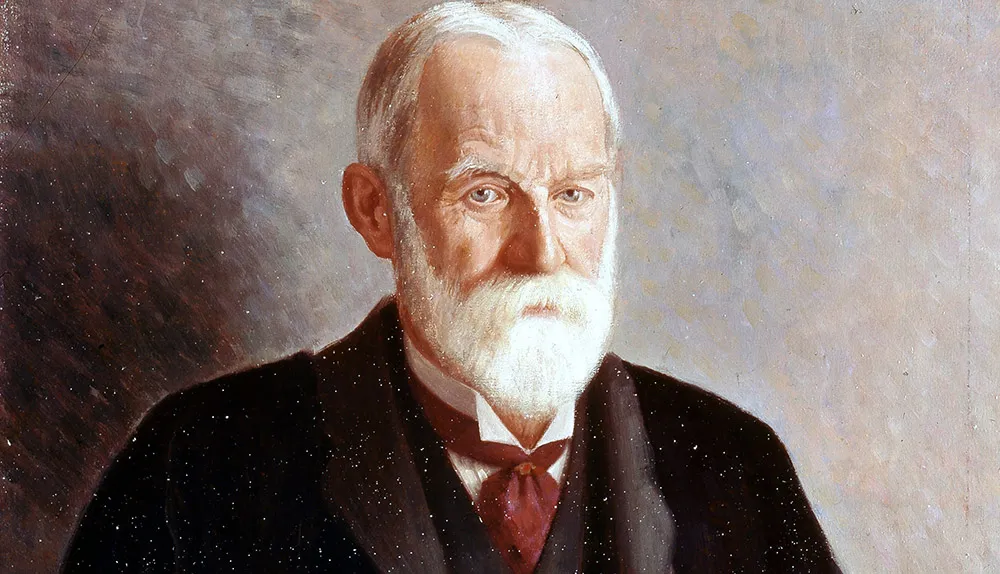
Darwin’s theory was inspired by the then-recent discoveries of the Moon’s outwardly spiralling orbit and the gradual slowing of the Earth’s rotation, both linked to tidal forces between the two. He argued that the young, semi-molten Earth might have spun so rapidly that the resulting tidal bulge around its equator became unstable, flinging off large amounts of material that then coalesced to form the Moon.
NEED TO KNOW
Isotopes
Two or more forms of an element that share the same chemical properties but have different atomic masses. The relative mix or ‘isotopic ratio’ found for particular elements varies widely across the Solar System, so this provides a useful clue as to where materials came from.
Planetesimal
A large body formed early in the history of the Solar System, with enough gravity to pull in gas and dust from the surrounding solar nebula and undergo rapid growth. Collisions between planetesimals are thought to have played a vital part in forming the planets.
Volatile
A chemical compound or element with a relatively low melting point that can be easily melted or evaporated by geological processes. The precise boundary between volatiles and high-melting-point substances varies depending on the context, but in lunar geology, volatiles include metals with melting points below 1,500°C.
Four years later, geologist Osmond Fisher added colour to the theory with his suggestion that the enormous Pacific Ocean basin could be the scar left behind by this separation.
Many doubted that Earth’s rotation could have slowed down quite so dramatically throughout its history; others pointed out that, big though it is, the Pacific basin has only a fraction of the volume of the Moon.
But the fatal flaw in Darwin’s theory emerged from an improved understanding of fluid dynamics. In 1930, geophysicist Harold Jeffreys showed that the enormous bulge that would have developed around Earth’s equator would also have acted as a natural brake.
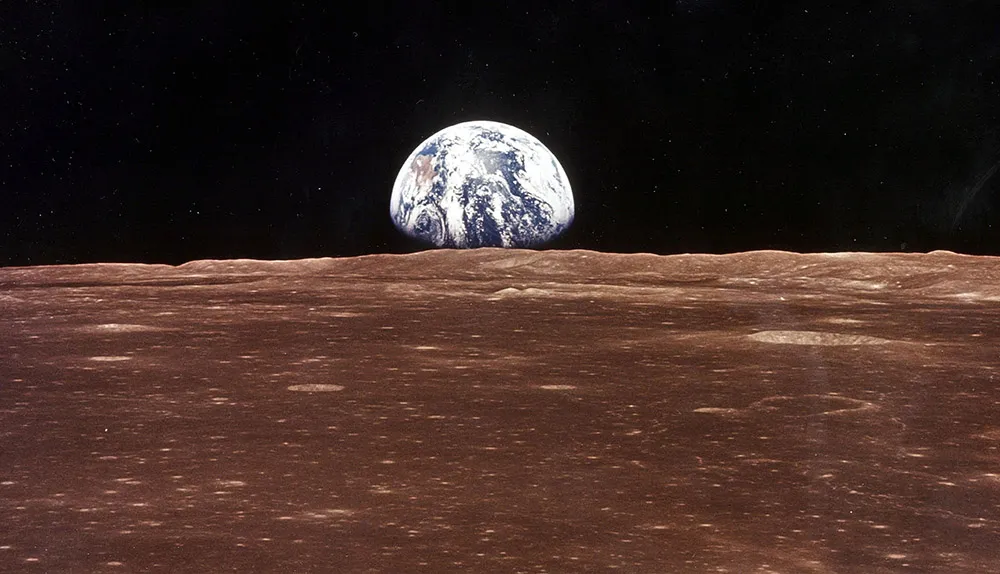
This would have prevented our planet reaching the extreme spin speeds needed to fling off the Moon. Variants of Darwin’s fission theory remained vague possibilities until the 1960s, but shortly after World War II they were faced with competition on three fronts.
In the early 1950s, chemist Harold Urey and astronomer Horst Gerstenkorn revived the capture theory first proposed by Thomas Jefferson Jackson See in 1909. In this model, the Moon evolved elsewhere in the Solar System, before being captured into a close orbit around Earth.
Some astronomers argued that the relative speeds of the two bodies during such an encounter would have been too large for gravity to overcome them, but a variant on the theory suggested that the Moon evolved in an orbit very close to Earth’s own, and so the capture was a slow, gradual affair.
Urey’s theory influenced NASA during the early planning of the Apollo programme, but so did the rival ideas of astronomer Gerard Kuiper. Kuiper revived the idea that the Moon had formed alongside Earth, showing how many of the problems due to the Moon’s large size could be overcome.

A third theory went almost unnoticed in the clash between Urey and Kuiper. In 1946, Canadian geologist Reginald Aldworth Daly had suggested a twist on Darwin’s fission idea. Instead of relying on a rapidly spinning Earth, he proposed that a major impact into the young Earth from another planet-sized body could have supplied enough energy to eject the material that formed the Moon.
But despite Daly’s reputation as a geologist, his musings on lunar origins were largely disregarded.
After Apollo
By the time Apollo 11 blasted off for the Moon in 1969, settling the dispute between the rival co-accretion, fission and capture hypotheses had become one of the Apollo programme’s key scientific aims.
The six manned lunar landings were deliberately targeted at areas of the Moon whose geological history was likely to be very different, and in total some 382kg of lunar rock was returned to Earth for analysis.
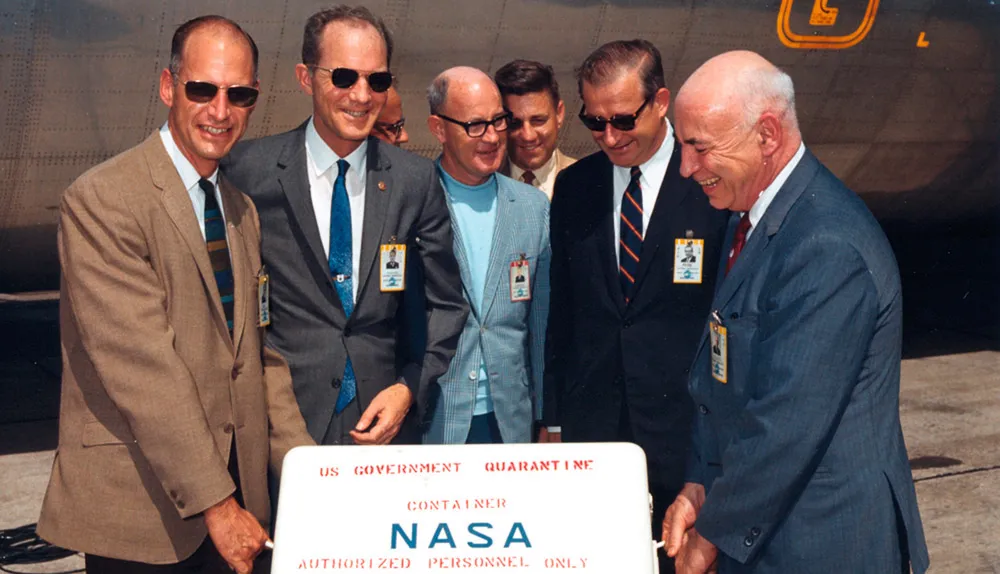
Each of the major theories had its own implications for the composition of lunar rock and the Moon itself. If the Moon formed alongside the Earth, or if the fission hypothesis was correct, twin bodies with very similar compositions would be created. A captured Moon, on the other hand, might be very different in its chemistry and mineralogy.
If the Moon formed at the same time, or was captured, it would probably be a ‘differentiated’ satellite, with internal layers and a dense iron core. But if it came from Darwin’s fission idea it might not be, since the material to form the Moon would come from relatively lightweight mantle rocks.
As the geologists got to work, they found the composition of lunar rock samples was an intriguing mix of the familiar and the unknown. Basalt rocks from the Sea of Tranquillity turned out to be heavily depleted of volatiles compared to those on Earth, but otherwise mineralogically very similar.

But what was most surprising was the widespread presence of the igneous rock anorthoside, and glass-like vitrified olivine. Both these and the lack of volatiles suggested the young Moon’s surface had been hot enough to develop an ocean of liquid magma.
In the early 1970s, supporters of the three main competing theories struggled to explain the Apollo data, but still none entirely matched the new evidence. Fortunately, an overlooked alternative was waiting in the wings – Daly’s giant impact hypothesis. Daly’s idea returned to the spotlight in a 1975 paper by William K Hartmann and Donald R Davis of the Planetary Science Institute in Arizona.
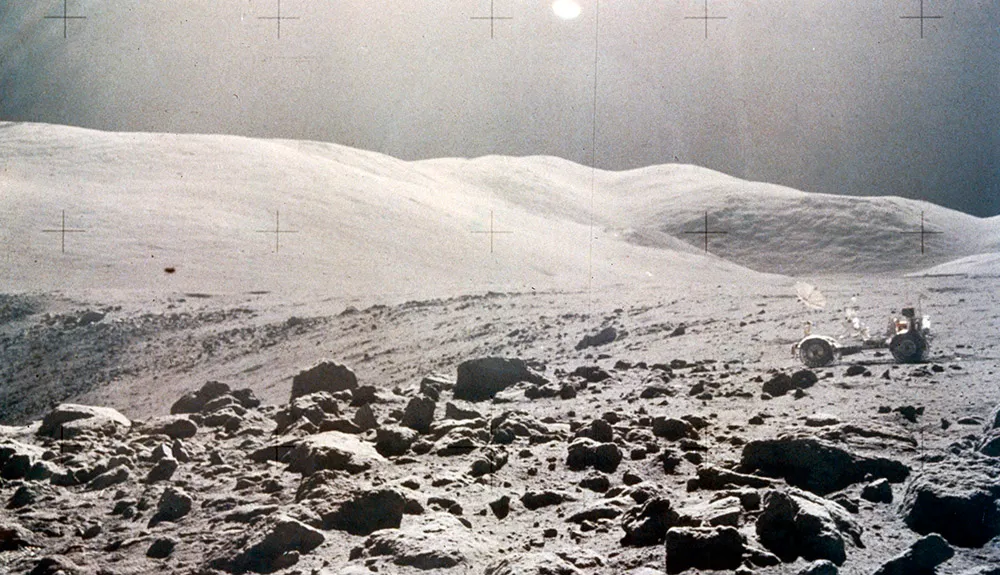
By this time the resurrection of Laplace’s nebular hypothesis was in full swing, thanks largely to the work of Soviet astronomer Victor Safronov. Safronov’s twist on the Laplace theory included a stage in which the early Solar System was littered with ‘planetesimal’ bodies ranging in size from hundreds to thousands of kilometres across.
Collisions between these formed the planets, and Hartmann and Davis were intrigued by the possibility that impacts by some of the smaller planetesimals onto the young Moon might have created the huge impact basins on its surface. Unaware of Daly’s earlier suggestion, they also hit on the idea that a larger planetesimal striking Earth could have powered a fission-like process, flinging a blend of rocks from Earth and the impacting body into orbit.
A year later, Alastair GW Cameron and William R Ward of the Harvard College Observatory suggested an impactor the size of Mars that struck the Earth at a shallow angle. This would ensure that the majority of ejected material came from Earth’s mantle, explaining the low density of the Moon compared to the Earth, and generate enough heat for most of the volatiles to escape into space.
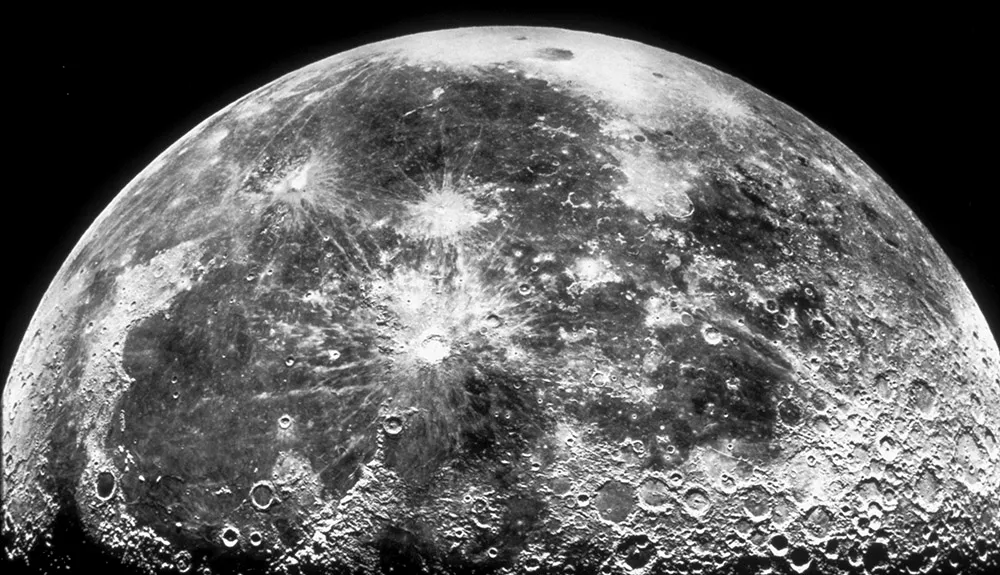
Hartmann and Davis’ theory dovetailed neatly with evidence from the Apollo missions, and gathered scientific momentum over the next decade. At the same time, the ‘big three’ theories were increasingly undermined by geological analysis and computer modelling. However, the complex nature of the Apollo results left plenty of room for debate, and it wasn’t until a 1984 conference at Kona, Hawaii that the idea really took hold.
The Moon today
Since the late 1980s, evidence that the Moon formed from material flung off in a giant impact has been mounting. Computer models have allowed the mass of the incoming body, the young Earth and the ejected material to be estimated, and in 2000 geochemist Alex Halliday even gave the impactor a name – Theia, after the mother of the Moon goddess Selene in Greek mythology – which seems to have stuck.
Theia is just one of a number of large hypothetical planetesimals that may have roamed the Solar System in its early days. For obvious reasons, it has exerted a peculiar fascination for astronomers and a considerable amount of effort has been spent on investigating its potential properties.
Read more about the origins of the Moon:
- A timeline of key discoveries
- The importance of lunar rocks
- The great scientists who uncovered the origin of the Moon
But, disconcertingly, as geologists have continued to learn more about the composition of the Moon, astronomers have once again been forced to revise the hypothesis in order to explain the mineral evidence.
It’s now clear that the Moon is not as dry as once thought, and that the water locked into some of its minerals is unlikely to have been dumped there by comets. The idea of a global magma ocean is no longer tenable and models must instead allow the Moon to coalesce without melting all the way through.
The isotopic ratios of certain minerals, meanwhile, are unnervingly similar to those found on Earth, with no signs of a contribution from the supposedly alien Theia.
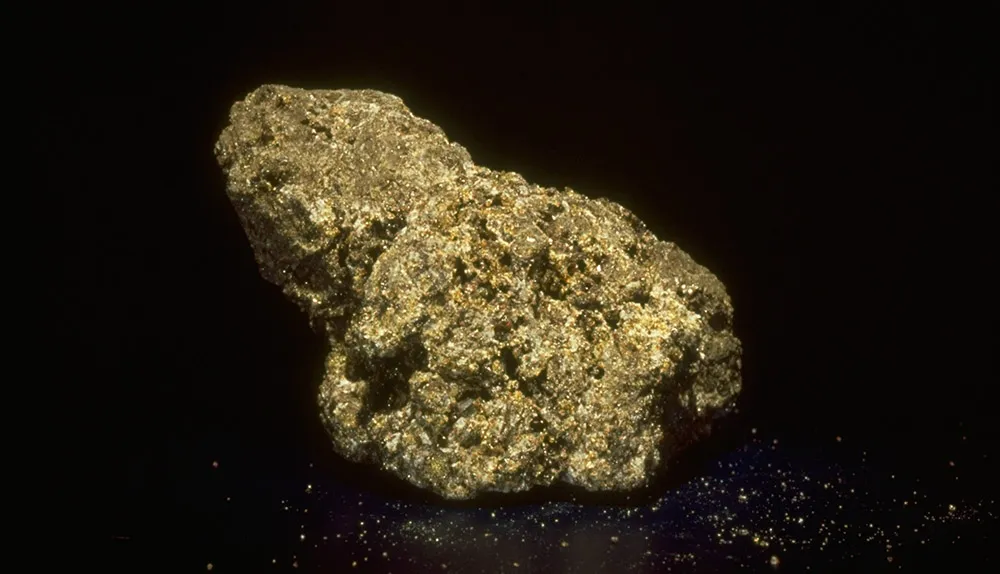
Three possible tweaks to the theory have emerged to explain these problems. One is that Theia evolved in the same part of the solar nebula as Earth itself, and therefore had near-identical chemistry. It may even have orbited Earth until it grew too massive and unstable.
A second idea is that Theia was much bigger than previously suspected – perhaps twice as massive as Mars – and so its impact substantially modified Earth’s own chemistry.
The third is that Theia was a stray ice dwarf world from much further out in the Solar System.
Its impact provided large amounts of energy to eject rock from Earth’s mantle, but its own volatile material evaporated away into space and it contributed little to the Moon’s eventual composition.
Despite these problems, it is clear that the giant impact hypothesis still provides by far the best explanation for the properties of the Moon as we see it today. It may need further amendments, but we’ve come an awfully long way in our understanding of our nearest cosmic neighbour.