Long perceived as the study of a bunch of irrelevant dead things, weare now seeing a radical transformation in palaeontology, the science of extinct life.
But the use of statistical methods to analyse big data, and the routine CT scanning of fossils to reveal their minute microstructures, have opened up entirely new fields of research, including how mammals became the warm-blooded milk-givers of the modern world.
Thanks to new technologies and big-data processing, knowledge of extinct life has exploded from the boundaries to which pen, paper and a keen eye had previously confined it. They reveal the origins of animals that define our planet, providing results used in everything from medicine to conservation and climate change mitigation.
Many of these methods are being deployed on fossils from the UK – such as the ones I work on from the Isle of Skye – contributing to wholesale revisions in our understanding of the evolution of major living groups, including our own lineage.
Using X-ray CT scanning (computed tomography) is a ubiquitous part of modern palaeontology. This is especially true for vertebrate animals, but it can be used for the study of invertebrates, plants and the rocks themselves.
Manual thin-sections are a long-established analytical tool in science, generated by slicing materials so finely that light can be passed through them. The advantage CT provides is a chance to observe the structure of fossils without damaging them.
Read more about palaeontology:
- New species of Triassic marine reptile discovered
- Prehistoric megalodon was a mega-shark that had 'fins as large as an entire adult human'
- Tiny beetle is the first-ever new insect species to be discovered in fossilised poo
The CT scan works on the principle of radiodensity: portions of the electromagnetic spectrum pass more easily through some materials than others. Materials that don’t allow electromagnetic radiation to pass through them are radiodense, those that do are radiolucent.
For most of us, our first and perhaps only encounter with CT is medical. In a conventional X-ray image of a broken bone for example, you see the bone as a flat, two-dimensional projection. It is extremely useful, but can only tell you so much. The CT scan achieves a three dimensional image by capturing multiple individual projections, like photographs from different angles, then applying algorithms to digitally reconstruct the whole object in the x, y and z axes.
The very first CT scan in 1971 took 180 individual images, each taking five minutes, and the reconstruction took two and a half hours. These days most CT scans palaeontologists acquire take less than an hour to complete – sometimes only minutes. It varies depending on the size and nature of the material being scanned.
Scanners are now so commonplace that it’s a relatively straightforward, inexpensive approach, permitting spectacular digital animation of fossil anatomy for use in science, as well as public outreach.
There is a new kid on the scientific block, increasingly employed as part of the palaeontologists’ toolkit. It’s called synchrotron scanning.
Synchrotron scanning is CT taken to the max, the superhero of visualisation. Materials that are hard to differentiate using conventional CT can be more readily discerned thanks to the intensity of synchrotron X-rays, which are better able to pass through materials and produce results faster. These X-rays have been peeled off electrons zooming near the speed of light, using magnets to direct them down tubes called beamlines, where they hit their target: the fossil specimen.
This form of scanning is being used for everything from understanding the structure of enzymes, to finding out why refrozen ice cream tastes bad (it’s the shape of the ice crystals). Synchrotron is also providing unprecedented new data on fossil organisms, including the mammals I study.

During my PhD, I began work on the skeletons of Jurassic mammals found in the 1970s on the Isle of Skye in Scotland. Despite being exceptionally rare, they hadn’t been studied before, remaining in a museum storage box for over 40 years. My first task was to obtain scans of their tiny bones. These animals were only palm-length in life, and their matchstick skeletons are still encased in hard limestone that can’t be removed easily using conventional techniques, such as dissolving the rock in acid.
But after three scanning attempts failed using regular CT at institutions across Scotland and England, I was running out of options. The blocks containing the fossils were too large, and those scanners weren’t powerful enough to penetrate them. Without detailed images I wouldn’t be able to reconstruct the skeletons or study their petite anatomy.
In the end, I took these fossils to the European Synchrotron Radiation Facility in Grenoble, where I worked with a specialist to obtain successful scans. Despite the futuristic technology, we placed the Skye fossils into cradles made out of bits of Styrofoam boxes, securing them with sticky tape and adhering them to the scanning platform on a bed of double-sided sticky tape. It was like Blue Peter does physics. A detector panel behind the specimen collected the patterns of shadow cast by the X-rays as they passed through, sending them to a computer to be knitted together.
In this way we imaged them in three dimensions, inside and out. It took 10 hours to scan the most important fossil at six microns in resolution – that’s six-thousandths of a millimetre, more than enough to do the job.
Read more about fossils:
- Lucy and Ardi: The two fossils that changed human history
- How to hunt for fossils in the UK
- Could there be fossils on Mars?
Synchrotron succeeds where conventional CT fails, saving the palaeontological day, but what do we do with the data we produce? The most obvious use for scan data is simple reconstruction of skeletons and other structures.
In 2018, I used CT data to examine a fossil mammal ear bone, or petrosal, that was smaller than a lentil. Ear bones are important in mammal evolution because they have an extraordinary range of hearing capabilities; from the highest echolocation of bats, to the deep belly-rumbles of elephants and blue whales. To understand how this audio range evolved, we need to look at ear structures through time.
Not only was I able to reconstruct this Jurassic ear bone, but trace the pathways of nerves and blood vessels snaking through it. This revealed how the soft tissues worked in the functioning ear of an animal that lived over 166 million years ago, providing another piece of the developmental puzzle.
The outrageous detail revealed by synchrotron scanning means we can look still deeper into old teeth and bones. The resolution is greater, and this allows researchers to investigate tiny objects in breathtaking detail.
In the last few years my colleague Elis Newham has been working with a team examining the growth rings in the teeth of ancient mammals to find out how long they lived. These rings, formed from a substance called cementum, mineralises in patterns of growth and cessation annually, just like in a tree trunk. They have been used by archaeologists to study ancient humans, but Newham and his team counted them in the first mammals – animals like Morganucodon, a mouse-sized ancestor from the Late Triassic of Wales.
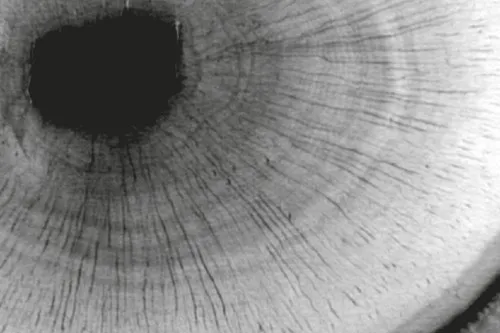
Newhams’s team found that the earliest mammals lived for over a decade, making them veritable ancients by small mammal standards. Little Morganucodon could have celebrated its 14thbirthday party, an unachievable milestone for most modern mammals of similar proportions. Only in the first members of modern lineages did lifespan significantly shorten to a handful of years.
This finding could hint at the timing of a major evolutionary change. The first mammals probably still laid eggs, and their long lifespan suggests they grew more slowly and maintained a slightly lower body temperature than most mammals do today.
The shortening of lifespans in the Jurassic may be linked to a rise in metabolism and resting body temperature; becoming ‘warm-blooded’. Newham and I are now using this technique on fossils from Scotland to see what secrets they might hold in their tiny gnashers.
So, if you still have that Indiana Jones or Jurassic Park picture of a palaeontologist in your mind, you couldn’t be more wrong.
Forget whips and guns, what real palaeontologists carry with them is their laptop. They may need a hat for occasional fieldwork, but most would do better investing in a comfy office chair because they’ll be tapping out most of their lives in front of a high-end computer. They type code like most of us compose text messages, and fetch CT scans like ordinary people grab takeaway coffee.
This article has been adapted from text in Dr Elsa Panciroli's new book, Beasts Before Us: The Untold Story of Mammal Origins and Evolution(£20, Bloomsbury Sigma).
- Buy now from Amazon UK, Bookshop.org and Waterstones