We’re in trouble. Our over-reliance on fossil fuels and our taste for foods with a high carbon footprint is causing disruptive climate change. Our throwaway society has flooded the land and seas with plastic pollution. And we face a growing public health crisis triggered by the rise of disease-causing microbes that we cannot kill with antibiotics.
Now for the good news. The last few years have brought promising evidence that we might be able to pull carbon dioxide (CO2) out of the atmosphere and slow the pace of climate change, that we have the potential to grow high-quality protein without the large carbon footprint, and that we can clean up our pollution and blunt the impact of antibiotic resistance.
The common element in all these potential breakthroughs? Bacteria. As unlikely as it might sound, our future health and happiness might be secured by these humble microbes.
1
Human health
Beneficial bugs that heal the body
Bacteria can be terrible for human health. They trigger deadly diseases including tuberculosis and cholera. As a consequence, bacteria-killing antibiotics have been called one of the greatest discoveries of the 20th Century: penicillin alone has saved an estimated 200 million lives over the last 80 years.
But bacteria can also be great for human health. Recently, we’ve learned that each of us carries trillions of beneficial bacteria and other microbes on our skin and inside our guts, known as the human microbiome.
Not only can they help us extract energy from our food, these ‘good’ bacteria sometimes protect us from disease-causing ‘bad’ bacteria. This is because the bad bugs can’t colonise our guts if the good bacteria take up all of the available space.
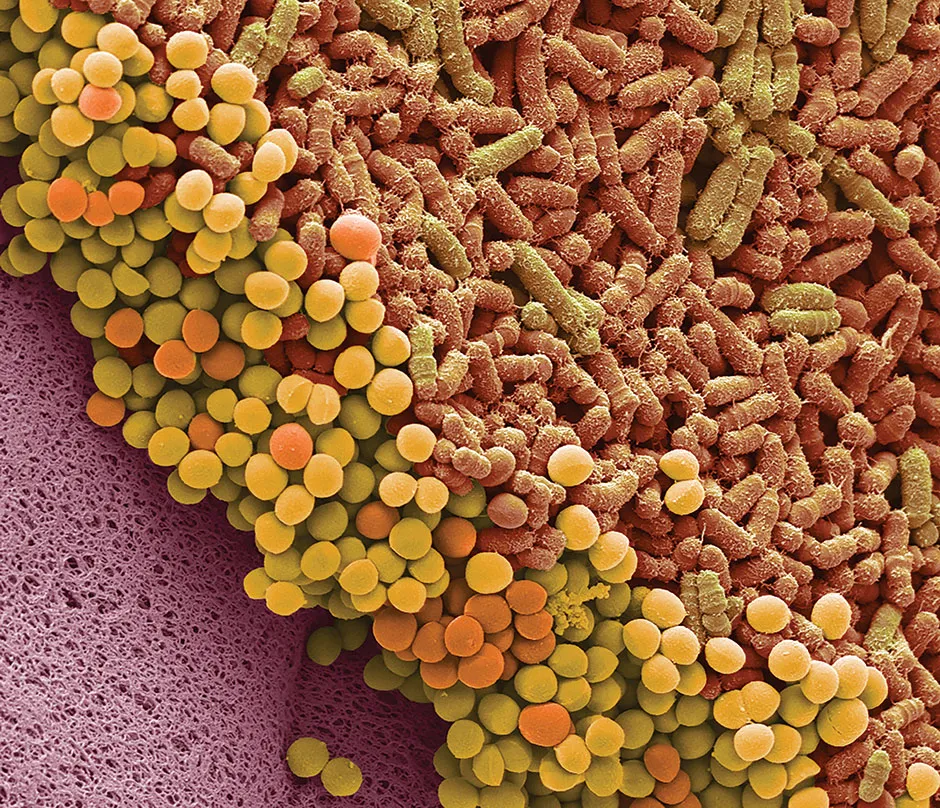
Or they do unless we disturb them. A downside of taking a prolonged course of antibiotics is that the drugs can kill off some of the good bacteria in our gut, offering disease-causing microbes like Clostridioides difficile an opportunity to take up residence there. C. difficile infections can cause diarrhoea, nausea and fever.
However, studies over the past couple of decades have highlighted a surprising way to treat C. difficile infections. If a sample of poo from a healthy volunteer is transferred into the diseased gut, the good bacteria it contains can outcompete the C. difficile growing there.
These ‘faecal transplants’ can help treat other diseases, too. But for the moment it isn’t clear exactly how. Prof Seth Bordenstein, from Vanderbilt University in Tennessee, studies the interactions between animals, microbes and viruses, and points out that we don’t know exactly which bacteria in the poo samples are responsible for the health benefits.
At the opposite end of the spectrum, there are bacteria we could use in medicine precisely because we know how they work. This is particularly true of Escherichia coli, a mostly harmless species that is at home in the human body.
Read more about the gut microbiome:
Researchers have learned so much about E. coli through the decades that it is now essentially a tiny, programmable biocomputer that could be used to treat disease.
“Some probiotic [health-benefitting] strains of E. coli can seek out and grow [inside cancer tumours], making them a suitable vehicle to deliver cancer therapeutics,” says PhD student Candice Gurbatri at Columbia University in New York.
Gurbatri is working with Prof Tal Danino, also at Columbia, to harness this tumour-seeking behaviour. They have inserted extra DNA into E. coli that allows the bacteria to manufacture potent cancer-fighting molecules called ‘nanobodies’.
Another snippet of extra DNA encourages E. coli cells to self-destruct once they have multiplied within the tumour, showering the cancerous tissue with the nanobodies. The end result is a form of E. coli that, when swallowed, will target and help destroy tumours.
Earlier this year, Gurbatri, Danino and their colleagues tested the approach in mice with promising results. But there will be regulatory hurdles to clear before human trials can begin. There are, understandably, ethical and safety concerns around the idea of treating people with genetically engineered microbes.
2
Renewable energy
The promise of bacterial biofuels
Bacteria are extraordinary chemists. Not only can they manufacture powerful anti-cancer drugs, they can also churn out renewable biofuels.
Earlier this year, Prof Nigel Scrutton at the University of Manchester and his colleagues published a study showcasing this potential.
They modified an enzyme called ‘fibroblast activation protein’ (FAP) that’s found in many bacteria, so that it breaks down human food waste through fermentation and generates propane gas. Propane is used in transportation fuel and in domestic heating and cooking.
Read more about renewable energy:
- The Orkney Islands: The energy revolution starts here
- Transparent solar cells could replace office windows
This on its own is a promising starting point for a commercially viable system. But Scrutton and his colleagues have boosted their chances of industrial success by inserting their enzyme into Halomonas bacteria, one of the few microbes able to survive in unusually salty water.
Most industrial fermentation systems are built from steel that must be carefully sterilised to make sure no rogue microbes enter the fermenter and reduce its efficiency. But as a Halomonas fermentation system uses salty water to operate, it saves on the need for this costly sterilisation because essentially nothing but Halomonas can survive.
“You could perform this fermentation even in a cheap reactor made out of a material like plastic,” says Scrutton. “It keeps the capital investment down.”

There are several other ways in which bacteria can generate biofuels.
One approach involves some unusual species of bacteria that eat and excrete electrons (negatively-charged subatomic particles), generating electricity. Stick an electrode in the ground in the right environment and a colony of these ‘electroactive’ bacteria will begin to grow around it.
Prof Derek Lovley at the University of Massachusetts and his colleagues have spent several years working out how to use these microbes for renewable energy generation.
In 2010, they took Sporomusa ovata bacteria and gradually starved them of their preferred ‘food’ – hydrogen – while also providing them with ample electricity.
Eventually, the microbes adapted to feed on the electrons instead of the hydrogen, and then used the electrical energy to convert CO2 into acetate, a commercially attractive salt that can be turned into biofuels or plastics.
Lovley calls the process ‘microbial electrosynthesis’, and it carries the potential to generate biofuels more efficiently than agriculture can. This is because the electricity to power microbial synthesis can come from solar panels, which are – perhaps surprisingly – a more effective way to capture solar energy than the photosynthetic plants, like rapeseed, currently used to make biofuels.
Earlier this year, Lovley and his colleagues revealed yet another way bacteria can help us produce sustainable energy. Some electroactive bacteria grow tiny electrically-conductive hairs: the researchers plucked off those hairs, sandwiched them between two electrically-conductive gold plates – and discovered the device generates electricity spontaneously.
Their ‘Air-gen’ device pulls electricity directly from the air, apparently taking advantage of differences in humidity in order to do so. Lovley and his team calculated that they could scale up Air-gen devices to outperform today’s commercial solar panels – and unlike solar panels, Air-gen would work at night too.
3
Water purification
Bacteria that feed on dirty water
Bacteria are the ultimate recyclers. While humans have traditionally looked on sewage as a waste product, bacteria have always treated it as food. This is why bacteria play a key role in wastewater treatment plants.
But running those plants can be expensive: we usually rely on oxygen-consuming microbes to break down the waste, which means wastewater must be kept aerated with air pumps that are costly to operate.
It doesn’t have to be that way, says Matthew Silver, co-founder and CEO at Cambrian Innovation in Massachusetts. Cambrian has developed an approach for cleaning industrial wastewater that generates more energy than it consumes.
Read more about clean water:
- How we can save the oceans, and how they can save us
- Toxic mercury pollution found in the ocean’s deepest point
This is possible, says Silver, because industrial wastewater is often full of energy. Wastewater from the dairy industry, for example, can be rich in carbohydrates and proteins. “What we’re treating – the complex carbohydrates – is basically like a fuel,” he says.
The company’s system largely relies on bacteria that can grow in the absence of oxygen, which saves the cost of running air pumps. In one part of the system, ‘electroactive’ microbes including Geobacter and Shewanella consume the waste in the water and excrete electrons and other charged particles.
Elsewhere in the system, so-called ‘methanogenic’ bacteria use some of these particles as a source of energy to convert CO2 into methane gas. “The methane can then be burned to generate heat or electricity,” says Silver.

The idea of using microbes to simultaneously treat wastewater and generate power goes back more than 15 years, but going from lab-based demonstrations to a commercially viable system has proved difficult.
“The energy density in sewage is low, so the volume you need to process to provide much power is enormous,” says Prof Buz Barstow at Cornell University, New York, who researches the potential for bacterial energy generation.
But Silver says the economics become more attractive when the technology is used at industrial sites, because industrial wastewater typically contains a higher volume of energy than municipal sewage.
4
Food
Protein from thin air?
Bacteria could feed the world. While some bacteria turn CO2 into valuable fuels, other bacteria – called ‘hydrogenotrophs’ – can transform CO2 into proteins suitable for human consumption.
In a sense, this shouldn’t come as a great surprise. The plants we eat, or that we feed to livestock, grow because they take energy from the Sun and use it to convert CO2 into carbohydrates. Strictly speaking, plants didn’t evolve this ability to transform CO2 into food: they gained the skill by incorporating photosynthetic bacteria into their cells.
Solar panels are more effective than photosynthetic organisms at capturing solar energy, and this raises an intriguing possibility. If we can take solar electricity and use it to help bacteria build protein from CO2, we could grow food more efficiently than ever before.
Read more about the future of food:
- The future of food: what we’ll eat in2028
- The artificial meat factory: the science of your synthetic supper
Solar Foods, a Finnish start-up, is one of several companies aiming to commercialise this non-photosynthetic food. Dr Pasi Vainikka, the company’s CEO, says the approach involves using power from solar panels to break down water molecules and generate hydrogen gas.
Solar Foods then feeds the hydrogen to bacteria growing in a fermenter – for commercial reasons Vainikka prefers not to reveal exactly what species of hydrogenotroph this is. The microbes use this hydrogen as an energy source to turn atmospheric CO2 into high-quality protein that could replace animal proteins in our diet.
“We are disconnecting food production from agriculture,” says Vainikka. Doing so has advantages. For instance, the fermenters that Solar Foods will be using in the coming years don’t take up much room. “We are basically operating a brewery,” he says.
Even allowing for the fact that the fermenters must be hooked up to solar power plants, the total land requirements are still small: Vainikka estimates that Solar Foods could produce a given amount of protein on one-twentieth of the land that a traditional farm requires.

This has the potential to allow some farmland to be converted to forest, which soaks up more CO2 than farmland does. “On a systemic level, we could be carbon negative,” says Vainikka.
Barstow says that research suggests there’s a huge amount of potential for creation of planet-friendly protein using bacteria and other microbes.
Vainikka envisions the Solar Food product as an additive to boost the protein content of foods like bread and pasta, or plant-based drinks such as oat milk.
And although the company – and others like it – may sink or swim on consumer attitudes, there are reasons to be optimistic that consumers may be willing to eat bacterial protein. After all, the UK-based manufacturer Quorn has sold microbially-generated protein for years – although it is made by fungi, not bacteria.
5
Climate change
Gobbling up greenhouse gases
Bacteria may be tiny, but they could have a huge impact in the battle to limit climate change. This is because many species of bacteria will ‘eat’ CO2, our principal greenhouse gas. But there is a flaw in this idea: bacteria only munch through CO2 slowly.
Faster-growing microbe species could remove more CO2, but those bacteria prefer to eat sugar over CO2. Last year, however, Prof Ron Milo and his colleagues at the Weizmann Institute of Science, Israel, showed that it was possible to turn a fast-growing microbe – E. coli – into a CO2 consumer.
Milo’s team modified E. coli by inserting DNA from CO2-consuming photosynthetic bacteria. Then they put the E. coli in an environment with high concentrations of CO2 and almost no sugar. After about a year, the E. coli had evolved to feed off the CO2.
Read more about climate change:
- Can we really achieve net-zero emissions by 2050, and what world would that would create?
- Why a carbon tax could be the answer to climate change
“Our work provides a proof-of-concept that dramatic changes in the metabolism of commonly-used microbes are attainable within reasonable time scales,” says team member Dr Shmuel Gleizer, also at the Weizmann Institute.
The E. coli gains the power it needs to eat CO2 from formate, a chemical that can be generated using renewable power from solar panels. In theory, says Gleizer, a solar panel-E. coli system could be 10 times more efficient than photosynthesis at removing CO2 from the atmosphere.
“I honestly think it’s a major breakthrough,” says Prof Uwe Bornscheuer, a biotechnologist at the University of Greifswald, Germany, who was not involved in the study. As the research develops, it could have an important positive impact on global warming, he adds.

Other scientists are experimenting with ‘probiotics for soil’ by injecting the dirt beneath our feet with carefully-selected microbes that both boost the soil’s carbon-storage capacity and accelerate the growth rate of crops planted within it.
Some researchers claim that, after application of soil probiotics, a hectare of farmland can store an additional 10 tonnes of CO2. This implies, they say, that the world’s agricultural land has the potential to absorb all of the carbon that humanity emits in one year.
But such research is controversial. The problem, according to Dr Adam Frew, an ecologist at the University of Southern Queensland, Australia, is that we don’t know enough about the nature of the microbial communities in soil, which makes modifying them in a beneficial way difficult.
“There are no simple shortcuts,” he says. Microbes may have the power to improve the carbon-storage potential of soil, but a better approach might be to achieve this by nourishing the microbes already living in the dirt rather than adding new ones.
6
Pollution
Cleaning up contamination
Bacteria will eat almost anything that contains carbon. Some microbes have even adapted to munch through oil spills and other forms of pollution. “There were big blooms of bacteria around the Deepwater Horizon oil spill [in the Gulf of Mexico in 2010],” says Prof Gautam Dantas, a microbiologist at Washington University in St Louis, Missouri.
This oil-eating ability has not gone unnoticed: researchers around the world are analysing the bacterial communities in soils near oil spills to identify which species are best at degrading the pollutant. The idea is that blends of these oil-eating microbes could be injected into the dirt anywhere in the world where oil pollution is a significant issue.
Read more about the BP oil spill:
- BP oil spill: 'invisible' pollution spread even further than believed
- Where will all the oil from Deepwater Horizon go?
A potential problem with this approach is that, as with the soil probiotics for crops, we don’t know a lot about the microbial communities within soil.
“Microbes added to the soil will probably struggle to compete against the indigenous microbes already there,” says Dr Hermann Heipieper at the Helmholtz Centre for Environmental Research, Germany.
But bacteria are still an important component in clean-up operations. The broader point to bear in mind is that microbes have an amazing capacity to evolve and adapt to consume new foods.

If the soil around an industrial plant becomes contaminated with oil, chances are that some of the microbes in that soil will begin eating it. We can help them consume their meal more rapidly by providing them with the other nutrients they need to remain healthy – an approach called ‘biostimulation’.
“This is regularly done by adding nitrogen, phosphorus and iron fertilisers,” says Heipieper.
It’s also possible to genetically engineer bacteria so that they convert heavy metals, such as mercury, into less toxic forms – but there are ethical and safety concerns about releasing genetically modified organisms into nature.
7
The plastic problem
For some bacteria, plastic is fantastic
Bacteria are not fussy. As well as oil, they’ll eat the refined, carbon-containing products that we manufacture from that oil. Like plastic, for example.
In 2016, a team in Japan went to a recycling site and collected samples of the plastic ‘polyethylene terephthalate’ (PET), which is used to make drinks bottles and the polyester in our clothes. They discovered that, on some of the samples, there were plastic-eating bacteria.
The microbes were using a pair of enzymes, dubbed ‘PETase’ and ‘MHETase’, to break down the plastic into smaller molecules such as ethylene glycol, which can be recycled to make new plastic. The researchers named the bacteria Ideonella sakaiensis.
Read more about plastic:
- Mark Miodownik: There is 'no value' in biodegradable plastic (at the moment)
- Meet the waxworm, a plastic-eating caterpillar that could solve our waste problem
What makes the discovery all the more astonishing is that PET plastic has been polluting the natural world for no more than 80 years. “It’s not long for this bacterium to have evolved [to eat] this novel, man-made carbon source,” says Bornscheuer.
I. sakaiensis eats plastic in the environment slowly, but biologists can help by isolating the plastic-eating enzymes inside the bacteria and tweaking their structure to make them work more efficiently.
In 2018, a team led by Prof John McGeehan at the University of Portsmouth engineered a version of the I. sakaiensis PETase enzyme that was almost 20 per cent more efficient at breaking down plastic. They are now trying to discover and engineer other bacterial enzymes that work efficiently enough for commercial use on a range of polluting plastics.
A study published earlier this year suggests success is possible. Researchers at French company Carbios took a bacterial plastic-eating enzyme – LCC – that was isolated from a compost heap about a decade ago, and tweaked its structure in the lab.

Normally, the enzyme breaks down PET plastic over the course of several days: the engineered version could do so in a matter of hours. The researchers then used the enzyme to break down waste PET plastic and turned the building blocks they produced into plastic bottles that were identical to ‘virgin’ bottles created from oil.
“This is a great proof-of-principle that enzymes can be engineered to help recycle some of our most polluting plastics,” says McGeehan, who was not involved in this study.
It’s not just PET plastic that bacteria will eat. Earlier this year, Heipieper and his team at the Helmholtz Centre for Environmental Research, Germany, found evidence that some bacteria may be able to break down polyurethane, used to make insulation and car parts.
“Reports are coming in from all over the world of bacteria that can degrade plastics – it’s really exciting,” says McGeehan.
8
Antibiotics
Can bacteria help undo the damage they created?
It was only in 1928 that Alexander Fleming discovered penicillin, the first antibiotic, and it was such an important finding that we still rely on these bacteria-slaying drugs to stay healthy.
But by using (and overusing) antibiotics, we’ve flooded the environment with them, which has given bacteria ample opportunity to evolve resistance to many of the drugs. Surprisingly, though, bacteria might be able to help us with the very problem they created, because some of them eat antibiotics.
“I’ve been working on this for a dozen years, but it’s still surprising to me that it happens,” says Dantas.
Read more about antibiotic resistance:
- Antibiotic resistance: Everything you need to know
- Is antibiotic resistance really as bad as climate change?
In 2018, Dantas and his colleagues worked out which enzymes help some soil bacteria to eat penicillin. They then transferred the ability into the super-common, mostly harmless, microbe E. coli.
It’s possible that such modified bacteria could eventually be used in wastewater treatment plants to remove antibiotics from the water – although Dantas points out there are significant hurdles to clear before that could happen.
Perhaps the greatest problem is that microbes swap DNA with other microbes they meet, so there would be real concerns that antibiotic-eating genes would pass into other bacteria, and ultimately into species that cause dangerous diseases. In that case, the harmful bacteria would have the ability to eat the drugs that are being used to fight them.
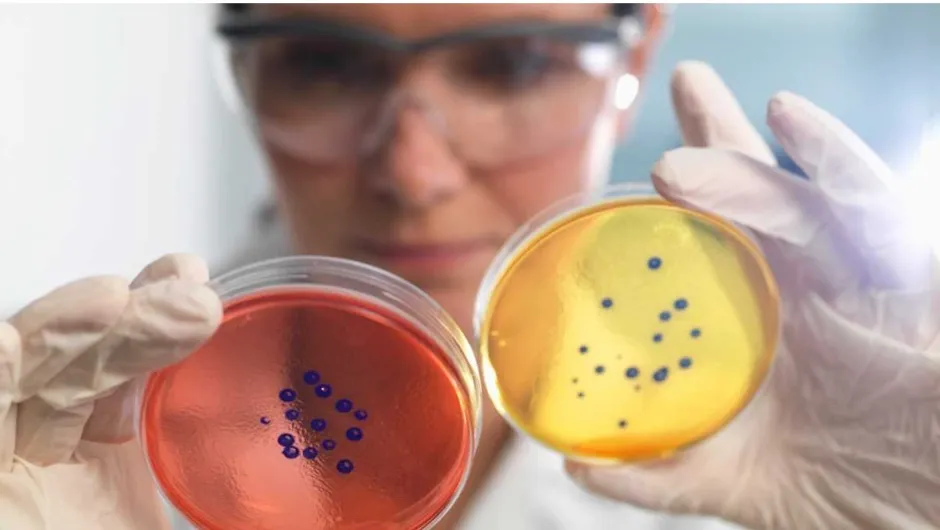
But given enough time to work with the bacteria, researchers may well find ways to overcome such obstacles and turn bacteria into a key weapon against antibiotic resistance.
The idea won’t surprise anyone who is aware of the astonishing potential bacteria have to overcome the many challenges we present them with – from destroying CO2, plastic and pollution, to creating food, clean water, renewable power and targeted cancer treatments.
- This article first appeared in issue 350 of BBC Science Focus - find out how to subscribe here