What is light? The question has fascinated scientists – and painters, poets, writers and anyone who’s ever played with a prism – since classical antiquity.
Pythagoras, Euclid and Ptolemy, for example, accepted that light moved in straight lines. But rather than assuming that light rays travelled from an object to the eye, they believed the eye emitted visual rays, like feelers, which touched the object and thereby created the sensation of sight in the mind.
Drop a needle on the ground, Euclid noted in the 3rd Century BC – search for it and you might wonder that the needle is not immediately visible. If light rays are emanating instantaneously from an object to the eye, he hypothesised that you should see it immediately.
He decided the reason for the delay must be that the visual ray, searching the ground, is yet to touch the needle. Then, in a flash, you spot it. This ancient idea of light and sight was taught in the West in various forms until the 12th Century AD.
It was rejected by Ibn al-Haytham, a 10th Century scientist from what is now Iraq. Al-Haytham was known in the West as Alhazen, with the nicknames ‘Ptolemy the Second’ and ‘The Physicist’.
He noted that you cannot look at the Sun for long without great pain; and if you stare at a bright object for half a minute and then close your eyes, a coloured image of the object floats into view. In each case, something emitted by the object must have entered the eye.
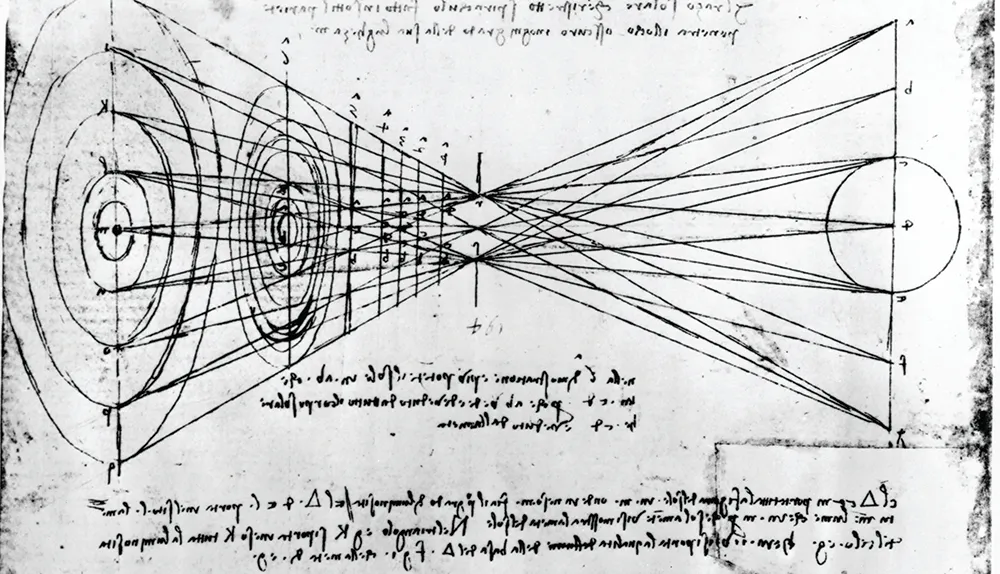
The later translation of Alhazen’s Book Of Optics into Latin led to its being read by, among others, artist Leonardo da Vinci, physicist Galileo Galilei, astronomer Johannes Kepler, philosopher René Descartes and mathematician Christiaan Huygens, all of whom contributed to our understanding of light and vision.
Da Vinci suggested that the eye is a camera obscura – literally, ‘a darkened chamber’ – into which light rays penetrate via a small aperture (the pupil) and create an inverted image of an exterior scene on the back of the eye (the retina). First adopted as a term by Kepler in 1604, the camera obscura became the dominant model of human vision in the 17th Century.
Read more about light:
- Why does light travel faster than sound?
- Why does light leave the position from which it is created?
- Do two mirrors facing each other produce infinite reflections?
Particle or wave?
By 1770, two theories of light, apparently incompatible, were in competition. The first, proposed by Huygens in 1678 and published in 1690, was an undulatory theory: light transmitted as waves. Light waves spread in all directions from a light source, and were detected by their creation of vibrations in the retina.
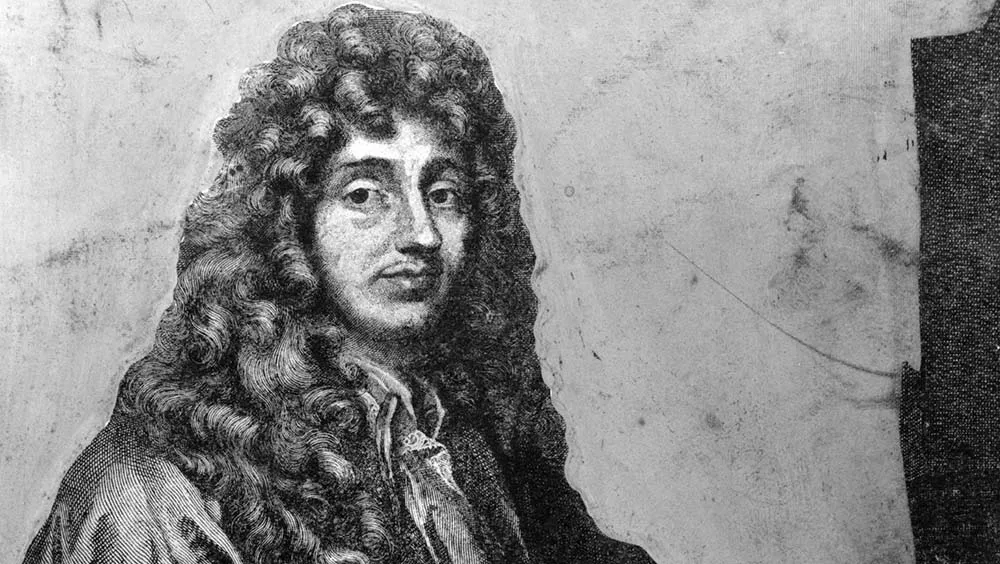
They were regarded as analogous to sound waves spreading from a tuning fork, with the retina rather than the tympanum as detector. With sound, the undulating medium was the air; with light it was supposed to be an invisible, mysterious medium known as ‘the ether’.
In contrast, Isaac Newton, who began his optical experiments in 1666, favoured a corpuscular theory: light as particles. Light rays spread from a light source in a stream of minute particles or ‘corpuscles’, shooting through empty space (rather than the ether) like bullets, and were detected by their impact on the retina.
That said, Newton was far from certain. He waited until 1704 before publishing his Opticks, and presented his ideas as a series of ‘queries’ with answers that, far from being definitive, occasionally favoured the undulatory theory.
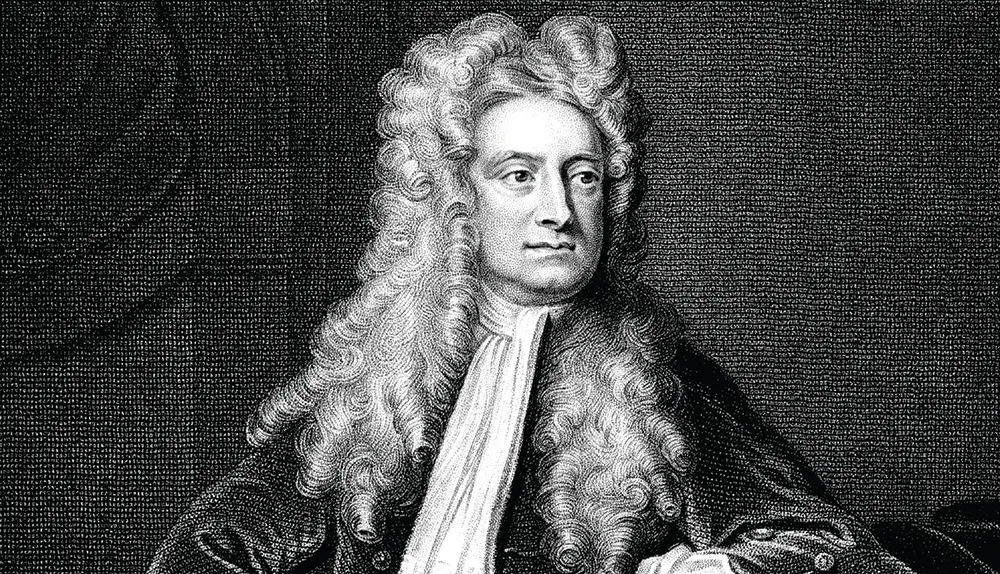
Of course, the real test of the two theories was experiment. How effective was each theory in explaining reflection, refraction and diffraction?
The simplest phenomenon was the transmission of light in straight lines, as in shafts of sunlight through a cloud or solar eclipses. Such behaviour was expected for a stream of corpuscles, but not for a wave.
Water waves rippling from a stone dropped into a pond could be seen to spread in all directions and bend around obstacles to some extent. Sound waves could be heard to bend.
Light, however, did not appear to bend. In Newton’s emphatic words: “Sounds are propagated as readily through crooked pipes as through straight ones. But light is never known to follow crooked passages nor to bend into the shadow.”
What about reflection? When light strikes a mirror, the angle of incidence is equal to the angle of reflection. In the corpuscular theory, the explanation was straightforward: the corpuscles must behave like billiard balls bouncing off the cushion of a billiard table at equal angles.
The undulatory theory, too, had no real difficulty. Once Huygens assumed that a light ray could be mathematically modelled as the path of a point on a wave front, he could easily deduce the law of reflection.
Refraction was a more decisive test. When light strikes the surface of water and passes through it, the angles of incidence and refraction differ.
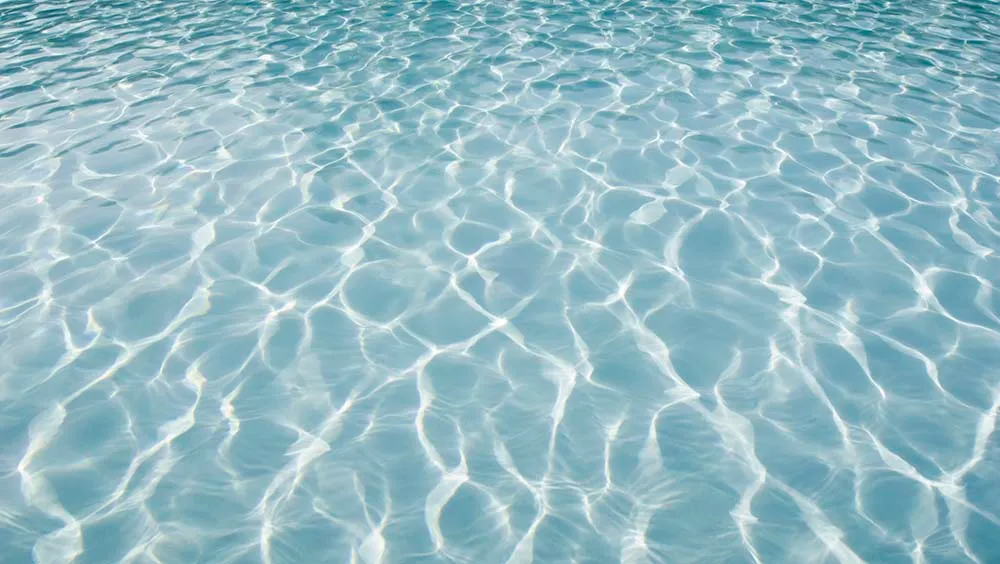
The angle of refraction is less than the angle of incidence, and so a ray is bent towards the normal (the perpendicular). Although their relationship had been formulated in 1621 as Snell’s Law, it still required a physical explanation.
Newton’s attempt was not very convincing. He proposed that light’s velocity in water was faster than in air – a counter-intuitive idea, given that water is a denser medium.
Once they entered the water, said Newton, the corpuscles were acted upon by a force which increased their velocity and altered the direction of their motion. However, the nature of this force was inexplicable, and it had no supporting evidence from other phenomena.
Huygens, by contrast, assumed that light travelled more slowly in water than in air. He then used the undulatory theory in a direct and simple way, without postulating any new force, to calculate Snell’s Law. But this verdict was by no means conclusive.
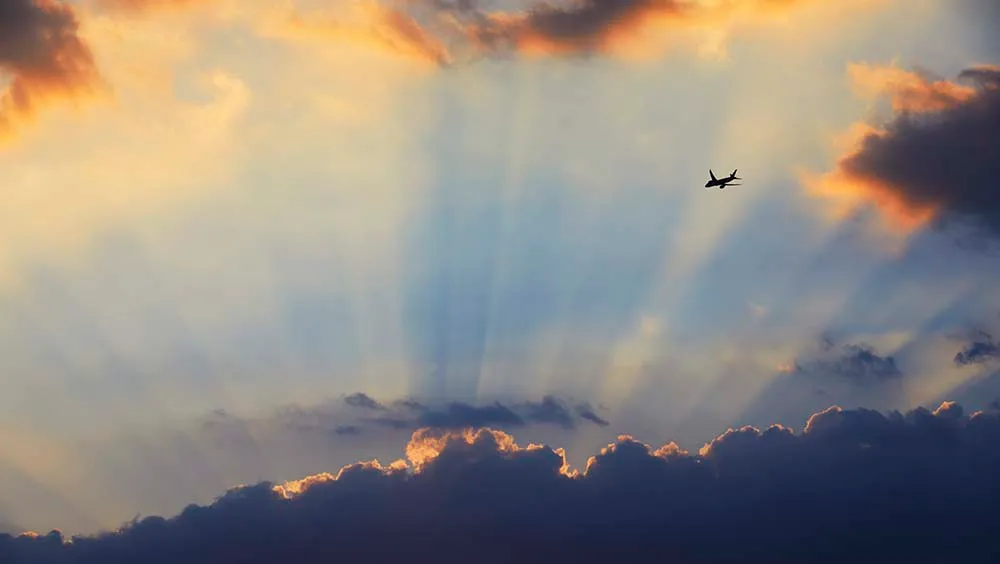
What precisely was the velocity of light in air and in water? Ole Rømer made the first modern estimate of light’s velocity in the 1670s using astronomical measurements, but not until 1850 was it measured accurately enough to prove that light moves more slowly in water than in air, as Huygens had assumed.
Finally, there was diffraction, a phenomenon first observed by Francesco Grimaldi in the mid-17th Century. He allowed a beam of light into a darkened room through a small circular aperture, then passed it through a second aperture and onto a screen. He noticed that the spot of light on the screen was slightly larger than the second aperture, and had coloured fringes.
When he placed a thin obstacle in the beam, its shadow was not sharp: there were bright bands, very narrow and coloured, following the outer edge of the shadow. In other words, light could be bent – diffracted – by apertures and obstacles, even if only very slightly.
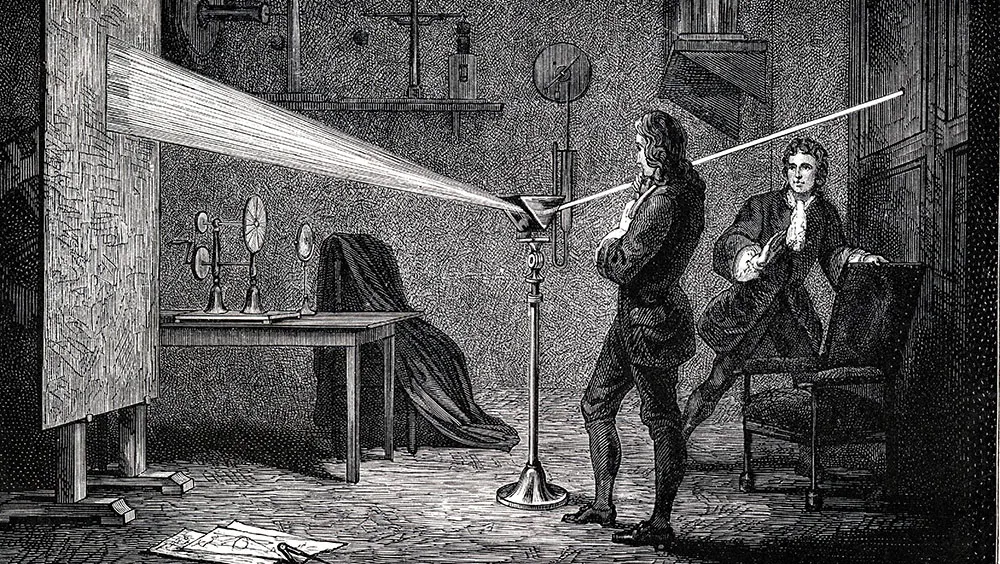
When Newton repeated Grimaldi’s experiments, he too observed coloured fringes. Perplexed, he claimed that the edges of an aperture or obstacle interfered with the paths of the corpuscles. Although the undulatory theory could not explain the colours, it explained diffraction better than its rival.
Nevertheless, Newton’s prestige allowed his theory of light to dominate the Age of the Enlightenment. In 1771, the first edition of the Encyclopaedia Britannica boldly declared: “Light consists of an inconceivably great number of particles flowing from a luminous body in all manner of directions; and these particles are so small, as to surpass all human comprehension.”
Not until the early 19th Century were corpuscles seriously challenged by undulations. Around 1804, Thomas Young demonstrated the interference of light, in which two overlapping beams of light produced bright and dark stripes, comparable to the interference of two water waves.
Corpuscles could not produce such patterns. Subsequent experiments on polarised light by French physicist Augustin Fresnel suggested a transverse light wave. Its components oscillate at right angles to each other and to the direction of propagation, as opposed to a longitudinal wave such as sound, which oscillates only in the direction of propagation.
A vibrating wave?
In the 1830s and 40s, Michael Faraday demonstrated the inter-relationship of electric and magnetic fields of force.
James Clerk Maxwell then took Faraday’s fields and merged them mathematically into a single concept: an electromagnetic wave with transverse electric and magnetic components, which Maxwell calculated would propagate through the ether at a speed similar to the measured speed of light, around 300,000km/s.
Different colours of light now corresponded to different wavelengths and frequencies. In the 1880s, German physicist Heinrich Hertz proved such waves’ existence experimentally.
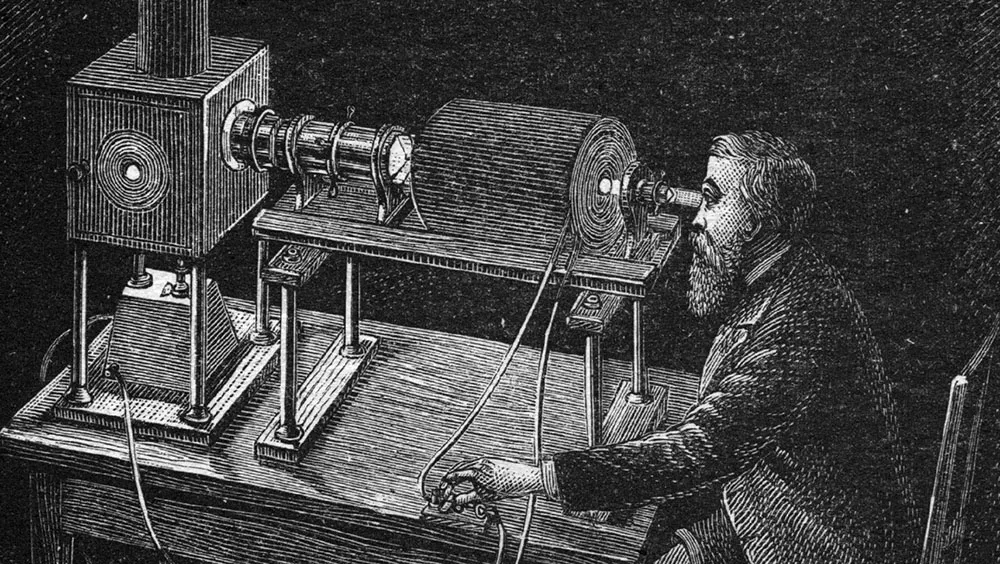
Maxwell solved one problem brilliantly, but offered no physical explanation for the ether. If light was a wave, what was vibrating? Persistent attempts to detect the ether failed during the latter part of the 19th Century.
After 1905, with the arrival of Albert Einstein’s Special Theory of Relativity, Maxwell’s ether was abandoned as an unnecessary concept. Relativity started with Einstein’s ‘thought experiment’ in 1895 about chasing a light ray.
Ten years later he wrote: “If I pursue a beam of light with the velocity c (velocity of light in a vacuum), I should observe such a beam of light as a spatially oscillatory electromagnetic field at rest. However, there seems to be no such thing, whether on the basis of experience or according to Maxwell’s equations.”
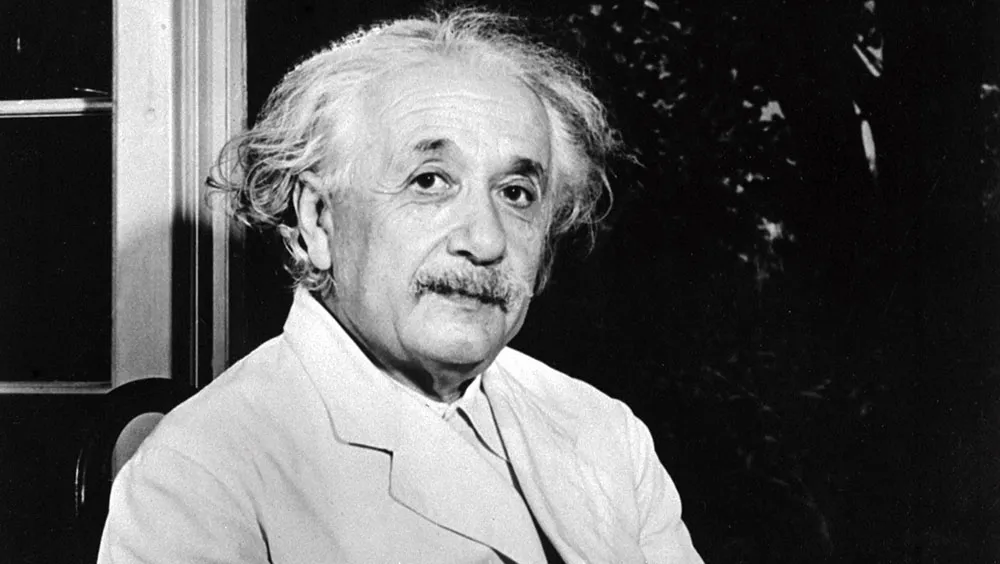
Were we to travel faster than light, Einstein imagined a situation in which we might run away from a light signal and catch up with previously sent light signals.
The most recently sent light signal would be detected first by our eyes, and then we’d see progressively older light signals. “We should catch them in a reverse order to that in which they were sent, and the train of happenings on our Earth would appear like a film shown backwards, beginning with the happy ending.”
What are photons?
The idea of catching or overtaking light was clearly absurd. Einstein postulated that the speed of light is always the same, independent of how the emitting source or the detector move, and without the need for any universal frame of reference such as the ether.
If this were not radical enough, in 1905 Einstein also proposed a comeback for Newton’s corpuscular theory in a more modern form. Instead of corpuscles, there were now light ‘quanta’ (later termed photons).
In 1900, Max Planck had postulated that the electromagnetic spectra from red-hot bodies could be explained only if radiation could be emitted or absorbed in discrete packets, or ‘quanta’.
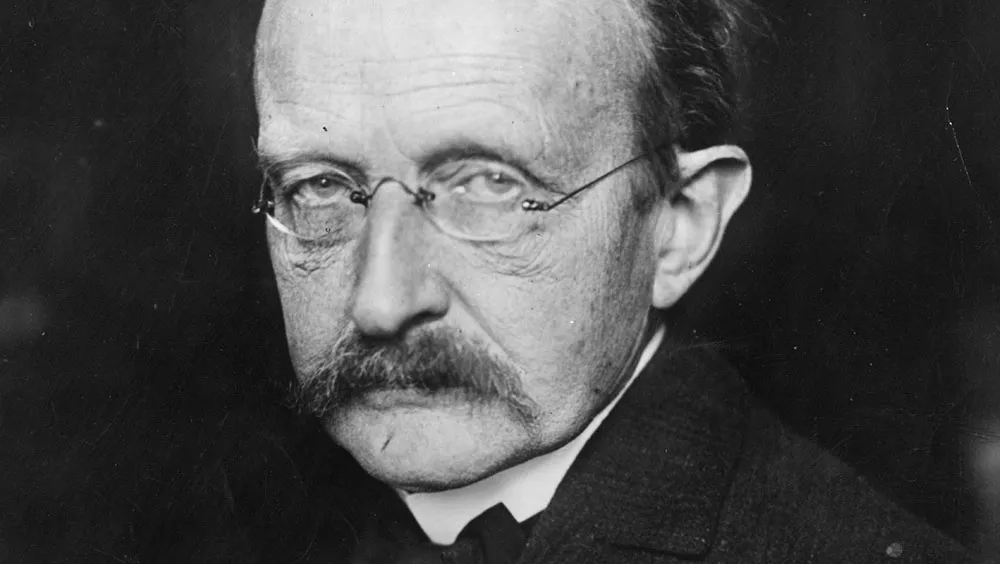
Now Einstein, after considering the photoelectric effect – the way in which certain metals emit electrons when exposed to radiation – proposed that not only were the emission and absorption of light quantised (formed into quanta), light itself was quantised. Between them, Planck and Einstein launched a theory that would determine the course of 20th Century physics.
Today, physicists are compelled to accept that light behaves as both a particle and a wave, depending on how they choose to measure its phenomena. But its underlying physical nature remains a puzzle.
A few years before his 1955 death, Einstein remarked: “All the 50 years of conscious brooding have brought me no closer to the answer to the question, ‘What are light quanta?’ Of course today every rascal thinks he knows the answer, but he is deluding himself.”
Since the 1980s, delicate experiments have replayed a version of Young’s key experiment and shown that a single photon can somehow interfere with itself. In his much-praised study Catching The Light, quantum physicist Arthur Zajonc admits: “Light remains as fundamentally mysterious as ever”.
- This article first appeared in issue 275 of BBC Science Focus Magazine–find out how to subscribe here
Read more about light: