Thirty light-years away, a star explodes. For several months, it shines 10,000 times brighter than the full Moon. It’s so bright that, during the day, it looks as if the Sun has been joined by another sun, pumping out a hundredth as much heat and light.
The good news is that you can sleep easy tonight, because this scenario would not happen. Life on Earth is safe from such an event. Superluminous supernovae – up to 100 times as powerful as any stellar explosion previously known – are not only very rare, but appear to detonate in galaxies quite a bit different from our own.
In 1931, Fritz Zwicky and Walter Baade, working at the California Institute of Technology in Pasadena, made an astonishing claim about exploding stars, or ‘novae’. Their work built on a discovery made eight years earlier by Edwin Hubble, who had used what was then the biggest telescope in the world (the 2.5m Hooker Telescope on Mount Wilson, which overlooks Caltech) to show that the mysterious spiral nebulae were in fact galaxies – great islands of stars separate from the Milky Way and millions of light-years away.
Zwicky and Baade noticed that sometimes such galaxies hosted stellar explosions, capable of outshining 100 billion normal stars. Knowing that such explosions were enormously further away than ones in our Galaxy, the two astronomers concluded that they belonged to a new class they called ‘supernovae’, around 10 million times more luminous than standard novae.
Read more about supernovae:
- Merging stars cause brightest supernova ever seen
- What ignites supernova explosions?
- Low-mass supernova sparked creation of our Solar System
What is a superluminous supernova?
The latest leap in luminosity is not as big as a factor of 10 million, but it is still impressive. A superluminous supernovae is about 10 times as luminous as a Type Ia supernova, which is powered by a star-shattering explosion of a white dwarf – a compact stellar remnant about the size of Earth – that has been swamped by matter from a companion star. And it’s about 100 times as powerful as a Type II supernova, the other main type of supernova, which is powered by the implosion of the core of a massive star at the end of its life.
The first superluminous supernova was discovered in 2005 and they were widely recognised as a distinct class of stellar explosion in 2011, principally by the work of Prof Robert Quimby of San Diego State University.

Their existence has come as a big shock to the astronomical community. “We thought we’d discovered all classes of exploding stars,” says Dr Matt Nicholl of the University of Birmingham. “How in the world did we miss the brightest ones?”
One reason superluminous supernovae went unnoticed until the 21st Century is they’re extremely rare, accounting for only about one in every 10,000 supernovae. The other reason is that supernova searches with telescopes tended to concentrate on big galaxies, with astronomers – quite understandably – reasoning that the more stars in a galaxy, the greater the chance of one going supernova.
Nature, however, had other ideas: it put superluminous supernovae into dwarf galaxies. “Only with the advent of robotic telescopes with wide fields of view were dwarf galaxies caught in our net,” explains Nicholl. “Once that started happening, we spotted superluminous supernovae. So far about 100 have been found.”
What causes superluminous supernovae?
What kind of stars are detonating as such cosmic mega-explosions? The biggest clue comes from the explosions’ spectra – the way in which the light varies with energy, or equivalent frequency. Astronomers can see the spectral fingerprint of heavy elements such as carbon, oxygen and neon, but not of the lightest two elements: hydrogen and helium. To grasp what this means, it’s necessary to understand something about the evolution of stars.
A star like the Sun fuses together the cores, or nuclei, of atoms of hydrogen to make helium, with the by-product being sunlight. But in stars that are between 8 and 25 times as massive as the Sun, conditions in the core can become dense enough and hot enough to fuse helium into carbon, carbon into oxygen, oxygen into neon, and so on. Potentially, such fusion reactions can proceed all the way up to iron, at which point they cease to generate any more heat (the hot gas of the core, no longer able to stop gravity from crushing it, promptly implodes).
The result is a star with an onion-like structure: the heaviest elements are in the core with each successive layer containing lighter elements, culminating in helium and finally hydrogen in the outer mantle. “Somehow the stars that detonate as superluminous supernovae have lost this hydrogen and helium,” says Nicholl.
The obvious way for a star to be stripped of its outer mantle of hydrogen and helium is via a stellar wind, similar to but far more powerful than the 1,000,000mph solar wind that blows from the Sun.
The problem is that stellar winds are stronger in stars that have a smattering of heavy elements mixed in with their hydrogen and helium mantle. Yet the low-mass galaxies in which the precursors of superluminous supernovae are located are deficient in such elements. This is basically because the galaxies’ weak gravities haven’t beenable to hang on to any heavy elements forged in earlier generations of stars and blasted into space by ordinary supernova.
Another way for a star to be stripped of its mantle of hydrogen and helium is if it’s in a close binary star system and the gravity of a massive companion star has stripped it off. “This seems the most likely possibility,” says Nicholl.
Where does the power come from?
The $64,000 question is of course: what powers these mega stellar explosions? An obvious possibility is that they’re merely souped-up versions of standard supernovae, whose power source is ultimately gravitational energy.
To understand gravitational energy, think of slate falling off a roof onto the ground. The slate’s gravitational potential energy (the energy it has due its height in Earth’s gravitational field) is converted into the energies of motion, sound and heat. Similarly, when the core of a star implodes, it’s like countless quadrillion slates falling, and results in a tremendous amount of gravitational energy that’s converted into a tremendous amount of heat. It is implosion, ironically, that drives explosion!
In a superluminous supernova, the spectrum reveals that between 5 and 20 solar masses of oxygen are ejected. In comparison, two to four solar masses of oxygen are ejected in a Type Ic supernova, which occurs in a standard star stripped of hydrogen and helium.

The implication is that the stars are only a few times bigger than the stars responsible for normal supernovae, and so a standard explosion is unlikely to make them 10 times as luminous.
The clincher for why superluminous supernovae are not simply souped-up versions of standard supernovae is that a normal supernova stays bright for a month or so because it’s powered by the radioactive decay of nickel-56 and cobalt-56, forged in the fury of the initial explosion. “However, something like 20 solar masses of such elements are needed to power a superluminous supernovae,” says Nicholl. “Though we see about 20 solar masses of oxygen, we don’t see an equivalent amount of nickel and cobalt.”
Another possible mechanism for a superluminous supernova involves the blast wave, expanding through space at about 10,000 kilometres a second, slamming into a slow-moving circumstellar shell of matter ejected by the star some time before the explosion. The rapid slowdown of the blast wave would shock heat the ejecta very efficiently, converting its energy of motion into prodigious amounts of heat and light.
“The problem is we don’t see any evidence of slow-moving stuff in the spectra of superluminous supernovae,” says Nicholl.
This leaves a final candidate for the engine of superluminous supernova. When the core shrinks, the endpoint is a highly compact object, such as a neutron star. Such an object, with a mass comparable to the Sun, but merely the size of Mount Everest, would be expected to be spinning fast, for the same reason that an ice skater who pulls in their arms spins faster: conservation of angular momentum. In fact, such an object could be spinning as fast as 1,000 times a second!
“Such an extraordinary flywheel has more than enough rotational energy to energise a superluminous supernova, if there is some way to transfer that energy outwards,” says Nicholl. “Fortunately, there is.”
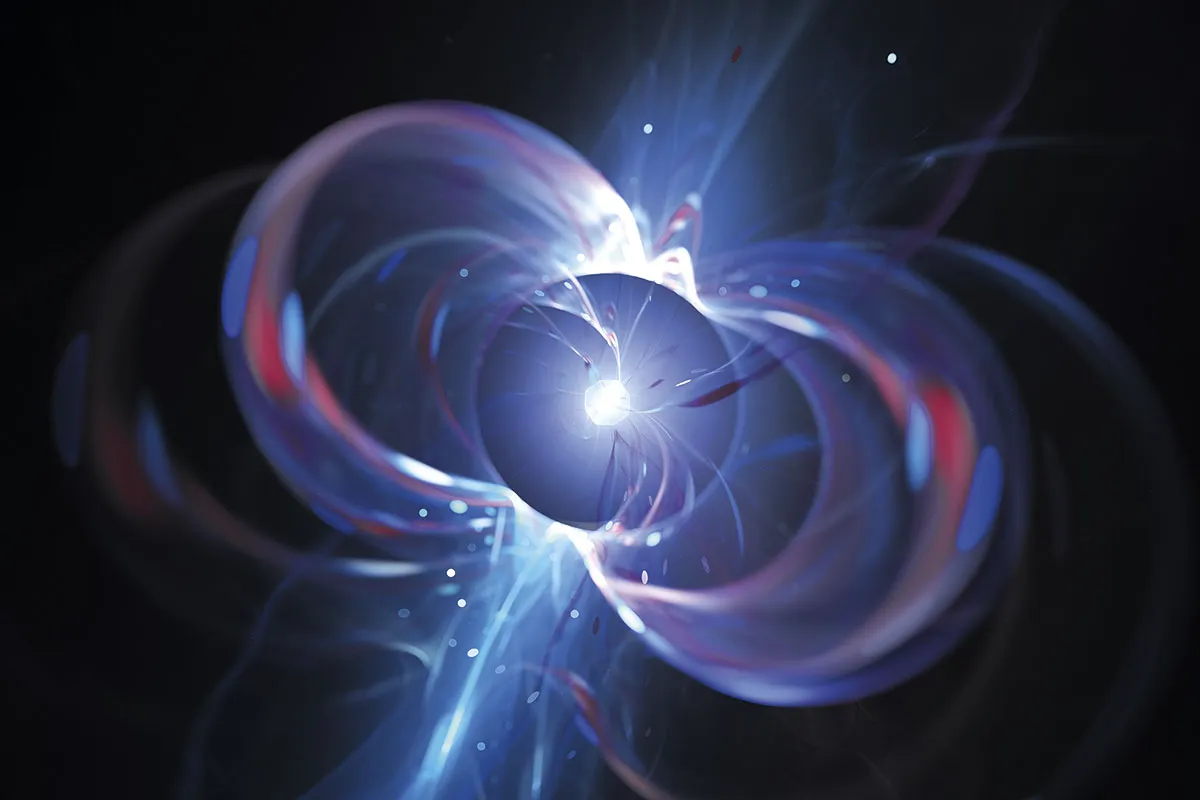
When the core of a star implodes catastrophically, any magnetic field that the star possessed is enormously concentrated and amplified. The neutron star may end up with a prodigious magnetic field – these neutron stars are known as ‘magnetars’. The magnetic field of such a magnetar could be in the range 1012 (a trillion) to 1015 (1,000 trillion) gauss (a unit that measures magnetic fields). For comparison, even the minimal field is 100 billion times stronger than a fridge magnet.
The problem is that the bigger the magnetic field, the more it interacts with surrounding material and the faster this interaction ‘brakes’ the magnetar’s rotation. “To keep a supernova bright for the month or so observed, a lower magnetic field is necessary,” says Nicholl. "There is a sweet spot at about 1013 to 1014 gauss.”
The precise mechanism by which the magnetar supplies energy to the material ejected by the star is not yet known. But Nicholl says there’s a way to prove or disprove the idea of a magnetar-as-central-engine. Its magnetic field is so strong it will conjure electron-positron pairs out of the surrounding vacuum, and their subsequent annihilation should create a distinctive spike of high-energy light, or gamma rays. “The falloff of gamma rays should precisely track the spin down of the magnetar,” says Nicholl.
“I think the magnetar model is the odds-on favourite for powering most superluminous supernovae,” says Quimby. “Some supernovae mark the births of neutron stars, and tapping just a small fraction of the energy from such beasts ought to be enough to produce some remarkable fireworks.”
But not everyone agrees that magnetars are the engines of superluminous supernovae. “I favour a mechanism where the ejecta from energetic supernova collide with massive circumstellar matter and the supernova kinetic energy is efficiently converted into radiation,” says Dr Takashi Moriya of the National Astronomical Observatory of Japan. But he concedes: “There may not be a single mechanism that makes supernovae extremely bright.”
Searching for superluminous supernovae
Although it has taken almost two decades to find the first 100 superluminous supernovae, the discovery rate will soon be boosted by the Vera C Rubin Observatory when it begins operating in Chile in October 2023. The telescope will observe the whole sky, night after night. “This ability will utterly transform the field,” says Nicholl. “Instead of 100 in 15 years, we’re expecting to discover 1,000 superluminous supernovae every year!”
An even more mouthwatering prospect will be provided by NASA’s James Webb Space Telescope, the successor to Hubble. With its 6.5m mirror (4.5 times the collecting area of Hubble), it will be able to detect superluminous supernovae at greater distances, which because of the finite speed of light, means at earlier cosmic times.
At the dawn of the Universe, there were many more dwarf galaxies in existence than now because they had not had time to merge to form the giant galaxies, such as the Milky Way, that we see today. They were also depleted in heavy elements because stars had not had time since the Big Bang to synthesise them. And there are theoretical reasons to believe that the first generation of stars to form after the Big Bang were monsters – possibly more than 100 solar masses. "Superluminous supernovae could easily have been more common at the beginning of time,” says Nicholl.
This raises an interesting possibility. The iron in your blood, the calcium in your bones, the oxygen that fills your lungs each time you take a breath… all of these were forged inside stars that lived and died, blowing themselves to smithereens, before Earth and the Sun were born. Perhaps superluminous supernova contributed a significant fraction of the heavy elements in the Universe. In which case, you may not need to look far to see the fruits of the superluminous supernovae. Just hold up your hand!
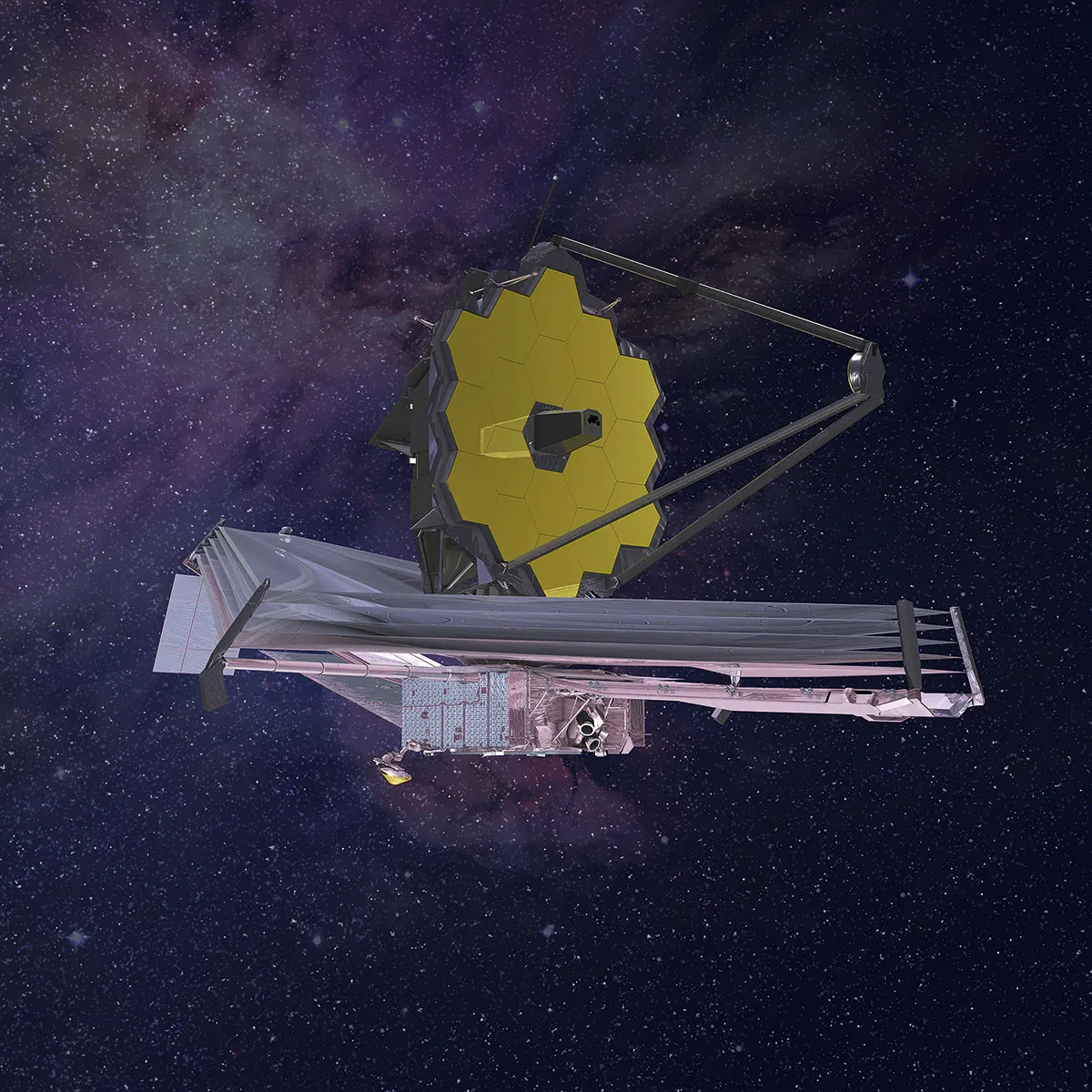
The first exploding stars were recorded by Chinese astronomers about 2,000 years ago. But it was not until 1931 that astronomers realised there was a class of super-explosions and not until 2005 a class of super-super explosions. The obvious question is: are there even bigger stellar explosions out there that we have so far missed? “I wouldn’t bet against it,” says Nicholl.
“Superluminous supernovae may mark the limit of what is possible for supernovae – at least locally,” says Quimby. “The big exceptions are the hypothetical pair-instability supernovae thought to exist only in the early Universe.”
In a pair-instability supernova, expected to occur in a star of between 130 and 250 solar masses, the interior gets so hot that gamma rays inside conjure into existence electron-positron pairs. These reduce the thermal pressure opposing gravity
trying to crush the core, triggering a catastrophic collapse and a titanic explosion that blows the star to smithereens.
A pair-instability supernova would shine 100 times brighter than even a superluminous supernova. Such supernovae might be detected by the James Webb Space Telescope. “As a hunter of exotic explosions,” says Quimby, “I like to think there are more surprises left to find in the Universe.”
- This article first appeared inissue 373ofBBC Science Focus Magazine–find out how to subscribe here
Read more about space: