In the 1970s, physicist Stephen Hawking tried to answer an apparently simple question: do black holes have a temperature? His analysis led to the concept which now bears his name: Hawking radiation. Not only did Hawking show that black holes radiate energy, he showed that they shrink incredibly slowly and eventually explode in a flash of gamma rays.
What is Hawking radiation?
The idea of Hawking radiation is based on the fact that empty space isn’t actually empty. This is perhaps a difficult concept to grasp. Although empty space contains no mass, no particles or quanta of energy, the quantum fields which define them still exist in the vacuum of space.
The usual explanation is that these fields, because they are not required to have zero energy, can create pairs of ‘virtual particles’, normally a particle-antiparticle pair that quickly annihilate each other. But near a black hole, the explanation goes, it is possible for one of those particles to disappear inside the black hole and be lost forever, while the other one escapes as Hawking radiation.
Discover more about black holes:
- What is a black hole and how did we discover them?
- If we made a powerful enough telescope, would we theoretically be able to see the light from the Big Bang?
- Are there any quantum effects that we can see in everyday life?
- Can life exist around a black hole?
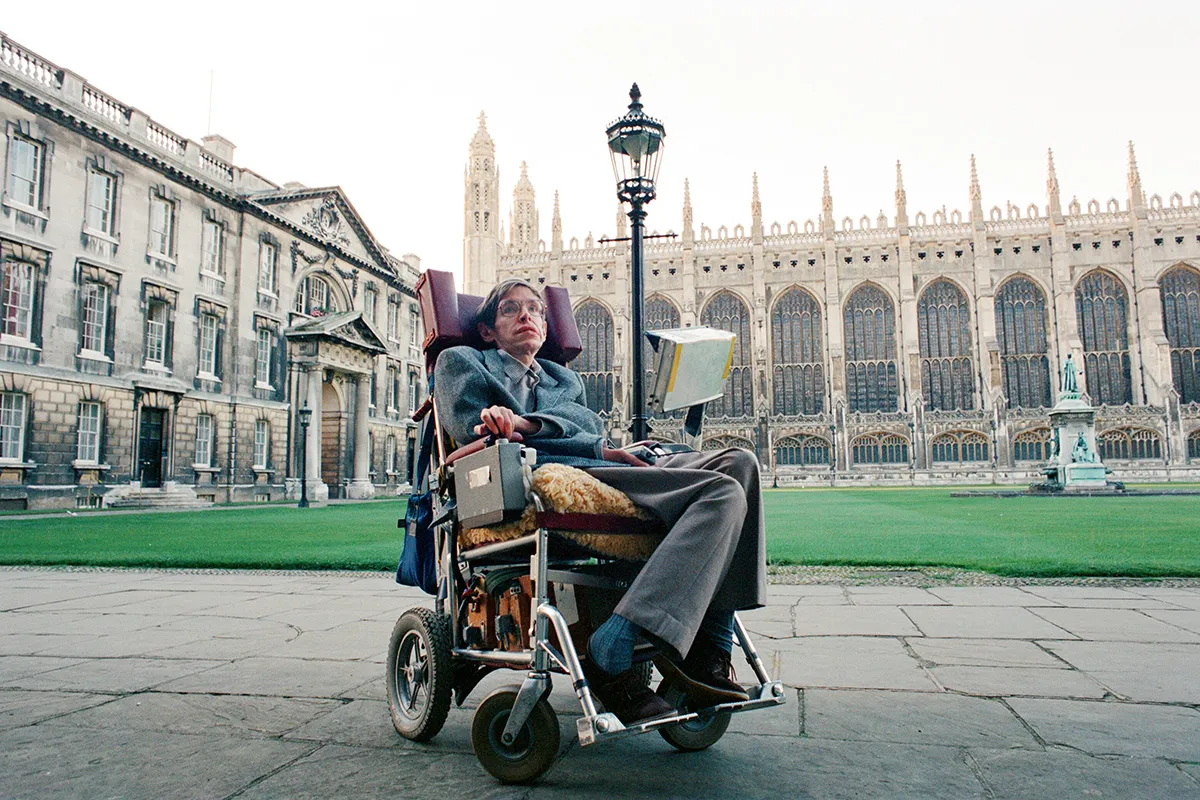
This explanation, although commonly used, is not entirely complete. Hawking radiation is actually the result of how gravity affects space-time, as described by General Relativity.
The quantum fields in empty space obey Heisenberg’s uncertainty principle, which means there is a limit to the certainty with which we can know their energy, or the time at which a specific energy can be assigned to them. Since a gravitational field bends space-time and affects the local passage of time, this means that regions of space-time with different gravitational curvatures cannot agree on the energy of the quantum fields. It is this difference in the energy of the vacuum at different locations in the gravitational field of a black hole that creates so-called ‘virtual particles’.
Can we detect Hawking radiation?
Hawking managed to answer his original question of whether a black hole has a temperature. They do, but those temperatures are extremely small. What’s more, Hawking showed that the amount of energy released by a black hole is inversely proportional to its mass. So, oddly, the higher the black hole’s mass, the smaller its energy release and temperature.
A black hole of one solar mass (one solar mass is equal to the mass of our Sun) might have a temperature of about 10-8K while a million solar-mass black hole would be about 10-14K. These temperatures, only marginally above ‘absolute zero’, are minuscule in comparison to the temperature of the Cosmic Microwave Background (CMB) – the relic radiation of the Big Bang which pervades all of space.
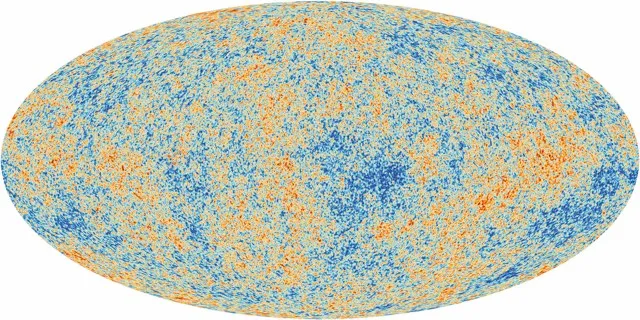
It also appears that the Universe cannot routinely produce black holes smaller than about 2.5 solar masses, so finding really small and hence hot black holes isn’t an option. It’s therefore likely that detecting Hawking radiation is virtually impossible.
There is one possibility though. Some astronomers hypothesise the existence of ‘primordial black holes’. These may have formed due to density fluctuations in the early Universe and may account for some of the mysterious dark matter that still eludes astronomers. Crucially, primordial black holes are not constrained by their size, so there is a chance that low-mass black holes may exist. These may emit sufficient Hawking radiation to be detected and, since their lifetimes are short compared to larger black holes, could reveal themselves in a flash of gamma rays during their dying moments.
Do black holes live forever?
One of the conclusions of Hawking’s work was that black holes don’t live forever. They eventually evaporate, in a very slow and mundane fashion. The release of Hawking radiation gradually reduces the mass of the black hole. So black holes that aren’t actively sucking in new material will slowly shrink and ultimately vanish.
The timescales for this evaporation are immense. For example, a black hole of one solar mass would take 1064 years to completely evaporate, whereas the age of the Universe is only of order 1010 years.
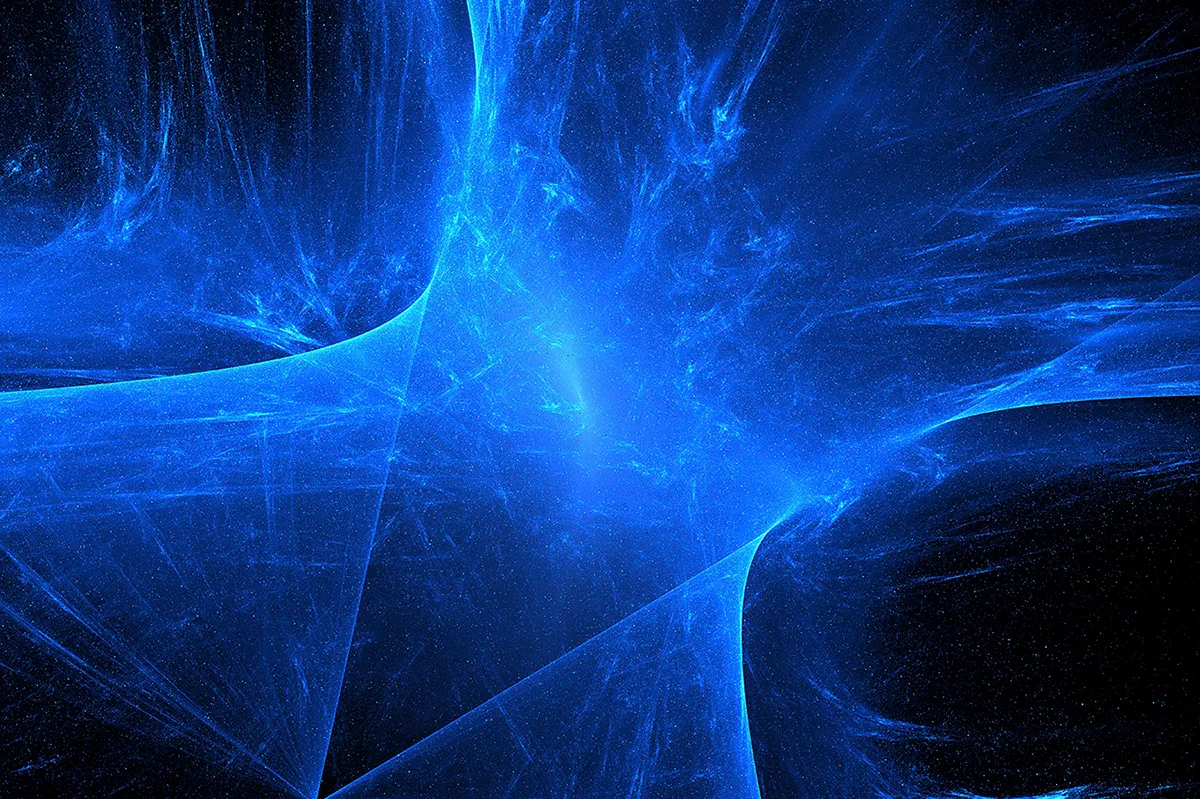
What is the black hole information paradox?
The evaporation of mass from a black hole due to Hawking radiation leads to a troubling problem known as the ‘information paradox’. One of the core principles of quantum mechanics states that ‘information’ cannot be destroyed. This means that, for example, if we have complete information about a system of particles, we can predict the future and the past states of that system.
The information held by particles that cross the event horizon of a black hole is forever ‘lost’ to us because it can never return. That isn’t a problem if the information remains intact within the black hole. The problem is that the black hole loses mass through Hawking radiation, but does not return that information to the accessible part of the Universe.
Eventually, the black hole disappears altogether and with it the information it has swallowed, violating the rules of quantum mechanics. The search for a resolution to this paradox has led to interesting new physics, but ultimately it may require a complete theory of ‘quantum gravity’ which, frustratingly, remains one of the unsolved problems of physics.
About the author -Alastair Gunn
Alastair is an astrophysicist and science writer at the Jodrell Bank Observatory, University of Manchester, as well as associate editor of the Royal Astronomical Society’s Astronomy & Geophysics magazine.
Read more:
- What are Lagrange points?
- What shape is the Universe?
- A Year on Mars: What has Perseverance achieved?
- Mystery of Jupiter’s polar cyclones solved using ocean physics
To submit your questions email us at questions@sciencefocus.com (don't forget to include your name and location)
- This article first appeared inissue 373ofBBC Science Focus Magazine–find out how to subscribe here